Advances in Animal and Veterinary Sciences
Research Article
Morphological Remodeling in Mouse Uteri Caused by Hormone Hypersecretion-Induced Increase in MMP Activity
Sang-Hwan Kim1, Jong-Taek Yoon1,2*
1Institute of Genetic Engineering, Hankyong National University, 327, Jungang-ro, Ansung, Gyeonggi-do, 17579, Korea; 2Department of Animal Life and Environment Science, Hankyong National University, 327, Jungang-ro, Ansung, Gyeonggi-do, 17579, Korea.
Abstract | Hormonal hypersecretion is important for the development and physiological function of ovary and uterus. PMSG, progesterone, and testosterone maintain the ovarian cycle of the uterus and regulate physiological mechanisms. However, how hormone hypersecretion causes uterine remodeling is unknown. we investigated uterine remodeling and MMP function in mice, induced by hormone hypersecretion after estrus synchronization. PMSG, progesterone, and testosterone were injected into six six-week-old mice for 14 d to induce hypersecretion. Evaluation of morphological remodeling of the uterus and expression pattern of MMP2 showed that hormone hypersecretion affected the endometrial cells and caused abnormal changes in the glandular cell zone. Notably, PMSG injection caused uterine hypertrophy. Normal MMP expression was observed; however, the expression of the MMP inhibitor TIMP was low. Progesterone did not affect uterine remodeling significantly and the uterus was unaffected by MMP and TIMP expression. However, testosterone caused morphological remodeling in the entire uterus, especially in the glandular cell zone, which is important for uterine function and physiology, and increased the MMP activity. Thus, hormone hypersecretion induced uterine remodeling by regulating MMP function. Additionally, testosterone caused uterine contractions by increasing the MMP activity, which is important for endometrial remodeling.
Keywords | Morphological, Hormone hypersecretion, MMP, Uterus, Mouse
Received | February 01, 2020; Accepted | April 15, 2020; Published | May 12, 2020
*Correspondence | Jong-Taek Yoon, Institute of Genetic Engineering, Hankyong National University, 327, Jungang-ro, Ansung, Gyeonggi-do, 17579, Korea; E-mail: [email protected], [email protected]
Citation | Kim S-H, Yoon J-T (2020). Morphological remodeling in mouse uteri caused by hormone hypersecretion-induced increase in MMP activity. Adv. Anim. Vet. Sci. 8(6): 582-589.
DOI | http://dx.doi.org/10.17582/journal.aavs/2020/8.6.582.589
ISSN (Online) | 2307-8316; ISSN (Print) | 2309-3331
Copyright © 2020 Kim and Yoon. This is an open access article distributed under the Creative Commons Attribution License, which permits unrestricted use, distribution, and reproduction in any medium, provided the original work is properly cited.
Introduction
Throughout the menstrual cycle, the uterus in females prepares for implantation and successful pregnancy after the proliferative and secretory stages. If embryo implantation does not occur, the endometrium regenerates and returns to the initial stage of menstruation. The ovary is considered to be the most important organ in the female ovarian cycle and the form of the uterus is determined by the action of the ovary (Hirshfield, 1991; Adriaens et al., 2004). In addition, the ovarian cycle is controlled by two important steroid hormones: progesterone (P4) and estradiol (E2); the concerted action of these two hormones plays an important role in the morphological and physiological changes of the uterus (Patel et al., 2015; Kumar et al., 2018). P4 affects reproductive function through receptors, including progesterone receptor A (PGRA) and progesterone receptor B (PGRB), and reconstructs the endometrium by maximizing the proliferation of the uterus and producing blood vessels such that implantation and pregnancy are possible (Rekawiecki et al., 2016). E2 also utilizes receptors, ERα and ERβ, and regenerates the uterus to the initial state of menstruation (Kumar et al., 2018). It is well known that hormonal imbalance generally causes polycystic ovary syndrome (PCOS) and increases complications in pregnancy, endometriosis, and venous thrombosis in the uterus, in addition to dysfunction of the ovary. These diseases affect 5–20% of women (Mahmood and Templeton, 1991; Surrey et al., 2002).
PCOS can cause abnormalities in the uterus. Many studies have shown that it is associated with the action of PGR and the abnormal expression of hormones (Margarit et al., 2010; Quezada et al., 2006; Hu et al., 2018). It was also reported that the occurrence of PCOS was increased by high concentrations of androgens (Kumar et al., 2005; Azziz et al., 2009). In other words, the morphological remodeling of the uterus depends on the secretion of hormones. Thus, it is important to understand how hypersecretion of hormones affects morphological remodeling of the uterus. Many studies have suggested that the dysregulation of MMPs in the uterus caused the development of endometrial neoplasms; however, studies on inflammatory or morphological changes in the endometrium have primarily focused on the action of MMPs in the outer membrane (Ishikawa et al., 2007; Vassilev et al., 2005; Kim et al., 2014). Thus, lesions and pathological abnormality in the uterus are caused by the imbalance of hormones, suggesting that an imbalance in MMPs and TIMPs may be useful for the diagnosis and treatment of PCOS and uterine dysfunction (Vincent et al., 2002; Bogusiewicz et al., 2007). Most studies on PCOS have focused on the effect of steroid hormones on MMP activity (Wingrove et al., 1998; Guccione et al., 2002; Huang et al., 2004). However, we have previously shown that hormonal hypersecretion may play a very important role in the expression of MMPs and in the morphological remodeling of the uterus. Therefore, the aim of this study was to determine the direct effect of MMPs on changes in the endometrial membrane by determining how hypersecretion of hormones, after ovulation, affects the activity of MMPs in the uterus and uterine remodeling.
Materials and Methods
Animals
Female ICR mice were obtained from Dahan Bio Link (Eumseong, Korea), all mice were housed within environmentally controlled temperature (26±1°C) at a 12/12 h (light/dark) schedule. All animal procedures followed the protocol approved by the Animal Experimentation Ethics Committee at Hankyong National University (permission number: 2018-1). All surgeries were performed under pentobarbital sodium anesthesia and every effort was made to minimize pain. All ovaries were treated to stimulate ovulation and then used in the experiment. Stimulation involved injecting 5 IU PMSG (Serotropin; Teikoku Zoki, Tokyo, Japan) into the abdominal cavity, followed by an injection of 5 IU HCG (Puberogen®; Sankyo, Tokyo, Japan) 48 h later.
Preparation of uterine tissue
Uteri were obtained from 18 mice at 6 weeks of age. Six female mice were randomly divided into groups, including the PMSG group, P4 group, and testosterone group. Uteri were also collected from six mice injected with PMSG, P4, and testosterone once every 48 h for 14 d.
Morphometric analysis
After the end of hormonal treatment, uteri from each group were collected and fixed in 70% diethyl pyrocarbonate (DEPC)-ethanol, dehydrated, paraffin-embedded, and sectioned at 5 um thickness. Thereafter, representative sections from each ovary paraffin-block, of different treatment groups, were randomly selected and stained with hematoxylin and eosin (H and E) for histological evaluation by using an optical microscope (40×).
Different variables measured in the D Score Equation
Glandular measurements
i) Volume percentage of stroma, which assesses the percentage of endometrial tissue composed of stroma (i.e., the inverse of glandular percentage, a measure of crowding). The volume percentage stroma equals 100 percent minus the volume percentage of glands.
ii) Gland outer surface density (OUTSD), which is a measurement of basement membrane length of the endometrial glands (measurement of gland complexity).
The formula for the calculation of the outer surface of endometrial glands is expressed as surface per unit volume (S/V), or surface density (mm2/mm3). Due to dimension reduction, such a surface is represented in the section as a line (mm), and the surface density, as surface line length per area (mm/mm2). OUTSD equals perimeter of endometrial glands in a field over the total area of that field.
Enzyme linked immunosorbent assay (ELISA)
For ELISA, ovarian protein samples were diluted in 100% assay buffer. VEGF (sc-7269, Santa Cruz Biotechnology, Santa Cruz, CA, USA) levels were measured using a quantitative sandwich ELISA kit (R and D Systems Europe, Abingdon, UK), according to the manufacturer’s instructions. All samples were measured in duplicate, and the mean levels were calculated for data analysis. The levels of hormones were determined by construction of a standard curve, which takes into account four parameters: (y = (A - D)/(1 + (x/C) ^ B) + D). The standard curve was based on seven known values. Average fold values for all hormones were measured and are expressed as mean ± standard deviation.
In situ zymography
To perform in situ zymography, deparaffinization/hydration was done twice by treating the samples with xylene, 100% ethanol, and 95% ethanol for 10 min, washing in DDW for 5 min, and boiling in 10 mM sodium citrate for 10 min. Thereafter, this was repeated 20 times on ice and thrice with DDW for 5 min. An emulsion of DDW, 10% SDS, and 2% glycerol and zymography reaction buffer were mixed at a ratio of 1:2 and placed on the slide, followed by enzymatic reaction at 37°C for 48 h in a slide box filled with 1 M Tris. After the reaction was complete, H and E staining was performed for histological evaluation using an optical microscope.
Immunohistochemical analysis
Sections of paraffin embedded ovarian tissue were used for IHC. Samples were sequentially treated with xylene, 100% ethanol, 95% ethanol, and DDW for 10 min each, and boiled in 10 mM sodium citrate for 5 min. Antigen sites on the protein were exposed by heating the sample in 10 mM sodium citrate (pH 6.0) at 95°C for 1 min. After rapid cooling to room temperature, endogenous peroxidase was visualized using 0.3% hydrogen peroxide in methanol. After three washes with 1X phosphate buffered saline (PBS), the slide was blocked with 1% goat serum and 3% horse serum for 1 h at room temperature. Incubation with primary antibody for MMP-2 (sc-13595, Santa Cruz Biotechnology) and TIMP-2 (sc-9905, Santa Cruz Biotechnology) was done overnight at 4°C. After a 5 min wash with 1X PBS, the secondary antibody was applied for 4 h at room temperature (25°C). Each sample was washed five times for 3 min each with 1X PBS. Thereafter, 300 μL of substrate (3,3’-diaminobenzidine, DAB) was added, for up to 10 min, and washed with DDW for 5 min. Counterstaining was done with periodic acid-Schiff reagent and hematoxylin solution (containing 4% acetic acid). Each sample was covered with the dehydrating and clarifying, Permount solution (Thermo Fisher Scientific, Waltham, MA, USA) and observed with an optical microscope (Nikon, Tokyo, Japan) at 200× and 400× magnification.
Western blotting
A total of 20 µg of protein was extracted from each sample and quantified using the Bradford assay (Sigma, St. Louis, MO, USA), according to the manufacturer’s instructions. Thereafter, the protein was separated by SDS-PAGE at 150 V, for 1 h, and transferred to polyvinylidene difluoride membranes (0.2 µm). The membranes were blocked with a blocking buffer containing skim milk for 1 h. The following primary antibodies were used: β-actin (sc-47778, Santa Cruz, California, USA), TIMP-2 (sc-9905, Santa Cruz, California, USA) and VEGF (ab2350, Abcam, Cambridge, UK), diluted 1:5000. The membranes were incubated with the primary antibodies at 4°C for 20 h and then washed three times (for 10 min each time) with TBS-T (1X Tris, 1X NaCl, and 0.05% Tween 20) to remove unbound antibodies. The secondary antibodies used were: anti-rabbit (ab6721, Abcam, Cambridge, UK) or anti-mouse (ab6798, Abcam, Cambridge, UK), diluted 1:5000. The membranes were incubated with the secondary antibodies at room temperature for 2 h and washed thrice with TBS-T for 10 min. Subsequently, the membranes were developed for 5 min using ECL reagents and exposed to X-ray film for 1 to 5 min. Protein expression levels were compared with that of β-actin and quantified using the Alpha Innotech ver. 4.0 program (San Leandro, CA, USA).
Zymography
To evaluate the enzymatic activity of MMP in mouse ovarian tissues, 20 mg of total protein, in a solution of 5% bromophenol blue, 10% SDS, and 2% glycerol, was added to 10 μL of FOZ loading buffer (5% bromophenol blue, 10% SDS, and 2% glycerol) and incubated for 5 min on ice. The proteins were resolved by electrophoresis conducted at 150 V for 1.5 h. After electrophoresis, the gel was induced twice with renaturation buffer (2.5% Triton X-100 and 1X PBS) for 20 min to renature protein and then washed with sterilized water for 20 min. Thereafter, the tissues were incubated at 37°C for 18 h in the zymography reaction buffer (1 M Tris-HCl pH 7.5, 5 M NaCl, 1 M CaCl2, 0.2 mM ZnCl2, 0.2% Triton X-100, and 0.02% NaN3). After completion of the reaction, the zymography induced protein was stained with Coomassie Brilliant Blue (Bio-Rad, Hercules, CA, USA) for 1 h and analyzed as previously described (Kim et al., 2014).
Statistical analysis
All data were collected, tabulated, and statistically analyzed using SPSS 22.0 for Windows (SPSS Inc., Chicago, IL, USA) and Med Calc 21 for windows (MedCalc Software bv, Ostend, Belgium). Statistical significance was tested at p < 0.05.
Results
Changes in mouse uterus caused by hormonal hypersecretion
Morphological remodeling of the mouse uterus, which was caused by hypersecretion of specific hormones after estrus synchronization, is shown in Figures 1 and 2. Hypersecretion of PMSG, P4, and testosterone caused morphological changes in the uterus. In the case of PMSG, the uterus was congested, the outer diameter was noticeably distended, and the length was shortened. HandE analysis showed that the endometrial thickness increased, the lumen widened, as the myometrium became thinner, and the development of perimetrium was also increased. In the case of P4, normal morphology was seen. The degree of congestion was lower than that of the PMSG treated group, the endometrium was less developed, and perimetrium development was normal. The lumen was also found to be of normal shape. In the case of testosterone, many differences were seen compared with the other two cases. The uterus had atrophied and was longer compared with the other cases, and the endometrium had atrophied. Overall, the luminal area was reduced and the perimetrium, which determines the outside of the uterus, was also reduced (Figure 2).

Figure 1: Anatomical and morphological changes in uterine tissues upon hormonal activation. (a–c) Morphological changes in the uterine tissue upon treatment with: (a) PMSG; (b) Progesterone and (c) Androgen. (a-1–c-1) Hematoxylin and eosin staining of the uterine endo/myometrium: (a-1) PMSG treatment; (b-1) Progesterone treatment; (c-1) Androgen treatment.
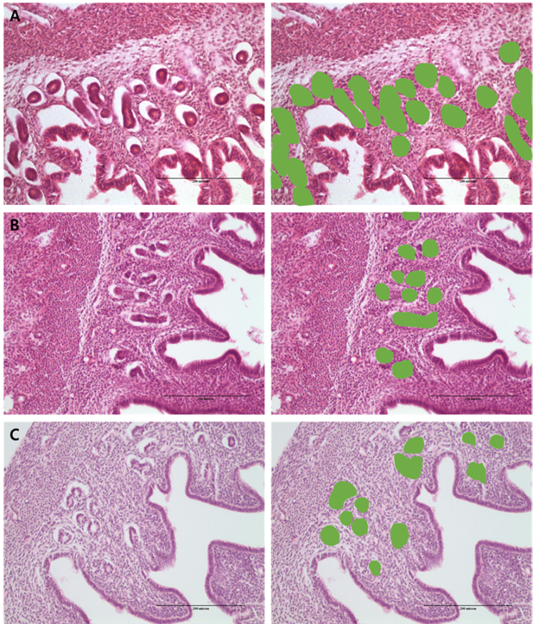
Figure 2: Morphometric measurement of % gland area using image analysis in the: (a) PMSG group; (b) P4 group and (c) Testosterone group.
Analysis of differences in endometrial development
The results from protein expression analysis, upon uterine proliferation, are shown in Figures 3 and 4. To evaluate the size of the proliferated endometrium, analysis of the glandular zone in the stromal cell section was performed. The size and distribution of the glandular zone were higher in the PMSG treated group, while in the testosterone treated group very little protein expression was seen and the cell zone was also very poor. In the case of P4, it was normal compared to the other groups and the endometrium was also normal. Thus, in the PMSG treated group, the endometrium was very dense, whereas in the testosterone treated group it was abnormal. Further, the expression of MMPs and hormone receptors, in the uterus of each treatment group, were analyzed. The expression of the follicular stimulation hormone receptor (FSH-r) was significantly higher in the PMSG treated group, while that of the luteinizing hormone receptor (LH-r) was higher in the testosterone treated group. The expression level of VEGF was similar to that of FSH-r. However, the expression of the MMPs which act on the apoptotic factor, Casp-3, and the change in the basal membrane of the cells were different from the results mentioned above. In summary, the expression of Casp-3, MMP-2, and MT1-MMP was relatively low in the PMSG treated group and was significantly higher in the testosterone treated group compared with the other groups (Figure 3). The activity of MMPs, analyzed by zymography, was very low in the PMSG treated group compared to other groups and in the testosterone groups it was relatively high, suggesting organizational changes in the testosterone treated group.
The activity and expression of MMPs in the endometrium
Results from the analysis of MMP activation in the uterine tissue of each treatment group are shown in Figures 4 and 5. To evaluate the difference, H and E staining and in situ zymography of the epithelial zone of the uterine endometrium and myometrium were performed. In the PMSG treated group, MMP activity and normal cell shape were seen in both sections. In other groups, the epithelium zone showed a higher level of MMP activation and the cell shape was seen altered. In particular, in the testosterone treated group, modified cells in both the myometrium and the endometrium were identified and the activity of MMPs was very high. The location of protein expression was very similar to that of the MMP active section. Notably, expression in the testosterone treated group was very high and that in the P4 treated group was very low, in all the sections. The levels of TIMPs which inhibit MMP activity seemed to be higher than expected in the testosterone treated group compared with the other groups, which indicates that the activity of MMPs and the expression of

Figure 3: Protein expression and ELISA analysis of FSH-r, LH-r, VEGF, MT1-MMP, MMP-2, and TIMP-2 in different hormone treatment groups of the mouse uterus. ELISA experiments were repeated thrice and data are expressed as average fold change (mean±SD). (a) Western blot; (b) Zymographic analysis of MMP-9 and MMP-2; (c) ELISA analysis. *, ** Different letters within the same column represent significant differences (p < 0.05).
MMP-2 were very high in the testosterone treated group, while in the PMSG treated group the expression of TIMPs was very low. In the P4 treated group, the expression levels of MMP-2 and TIMP-2 were similar.
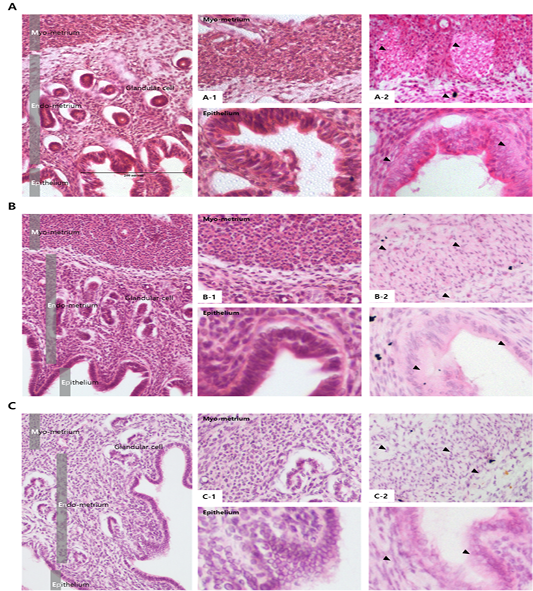
Figure 4: Analysis of MMP activation in different hormone treated groups of mouse uterine tissue. Black arrows indicate cells positive for MMP. Corpus luteum cells were counterstained with hematoxylin. Magnifications for the larger and smaller panels are ×200 and magnification x400 respectively. (a) PMSG treatment; (b) Progesterone treatment; (c) Androgen treatment; (a-1–c-1) Hematoxylin and eosin staining of the myometrium (upper panel) and the endometrium (lower panel), (a-2–c-2) In situ zymography analysis of the uterus.

Figure 5: Immuno-localization of MMP-2 and TIMP-2 in the uterine tissue of different hormone treated groups. Black arrows indicate cells positive for MMP expression. Metrial cells were counterstained with hematoxylin. (a) MMP-2 localization; (b) TIMP-2 localization. (a-1–b-1) PMSG group; (a-2–b-2) P4 group; and (a-3–b-3) Testosterone group.
Discussion
Hormones play an important role in the formation and the development of the uterus, and the action of hormones in the uterus changes the uterus in a timely manner, leading successful implantation and the return of estrus (Kumar et al., 2018). In other words, changes in the uterus are determined by hormonal action, but their effects on the external changes in the uterus are not known compared to the effects of hormone hypersecretion on PCOS (Quezada et al., 2006; Hu et al., 2018). This study was performed based on the hypothesis that hormone hypersecretion may lead to morphological remodeling in the uterus and the increase in the activity of MMPs (Vincent et al., 2002; Bogusiewicz et al., 2007). After the estrus of six-week-old mice was synchronized, PMSG, progesterone, and testosterone were injected into the abdominal cavity, every 48 h, to maintain high levels of the hormones in the body for a certain period and morphological changes in the uterus were observed for each hormone. Progesterone was expected to thicken the endometrium and change the proliferative state of the uterus to facilitate implantation, by changing the cytoplasm rapidly (Rekawiecki et al., 2016; Cousins et al., 2014); however, only a normal uterus was formed. However, in the PMSG treated group different results were obtained. It has been reported in many studies that PMSG causes polycystic ovary syndrome and that the hormones have a significant effect on the formation of follicles (Rebar et al., 1976; Cho et al., 2006).
However, in this study, an increase in PMSG resulted in the development of abnormal forms of the uterus. Notably, endometrial development was much higher compared to other groups. The expression of FSH receptors and the angiogenesis factor, VEGF, were high, while the activity of MMPs was not very high. This is somewhat different from the findings of Chen et al. (2019), who reported that PMSG had positive effects on the formation of endometrial vessels (Chen et al., 2019), whereas hypersecretion results in the maximization of the uterus and abnormal endometrium hypertrophy. Progesterone treatment might be associated with the proliferation of the uterus, which also seemed to play some role in PCOS. In addition, in a previous study, PGR has been shown to be abnormally expressed in the rat uterus with PCOS (Quezada et al., 2006), and the form of the uterus seemed to be changed by the action of progesterone. Thus, hypersecretion of progesterone may reconstruct the form of the uterus, but has little effect on the form of the uterus. Omar et al. (2019) reported that P4 controlled the expression of BCL-2 protein and inhibited apoptosis of endometrial cells (Omar et al., 2019). In this experiment, however, the normal form of the uterus was observed. Therefore, P4 maintains normal morphological form after uterine remodeling (Kim et al., 2014).
In this study, we focused on the changes in the uterus caused by the hypersecretion of testosterone. In women, testosterone secretion is one of the most important features of PCOS, which is mainly caused by excessive androgen production in the ovary, but can also be caused by androgen production from the adrenal glands (Kumar et al., 2005). Studies of role of testosterone in the formation of the uterus are very few. Testosterone, which is a hyperandrogen, has a direct role to play in the endometrium and may affect tissue remodeling during menstruation, pregnancy establishment, and proliferation of endometrial cells (Milne et al., 2005; Simitsidellis et al., 2018). These findings suggest that testosterone can play an important role in the morphological changes of the uterus. In this study, testosterone caused uterine contraction and caused abnormally shaped cellular layer of the endometrium. In particular, the activity of MMPs was higher compared with other hormone hypersecretion groups, which seemed to affect the entire uterus and to regulate the normal function of the uterus. Hypersecretion of testosterone is thought to affect the remodeling of the uterus by increasing MMP-2 expression in the uterus, and the expression of Casp-3, the apoptotic factor, was also seen to be increased, which caused abnormal changes in uterine physiological functions. This result is similar to that of Zang et al. (2008), who reported that testosterone caused uterine contraction (Zang et al., 2008). However, they suggested cell reactions in the endometrium after menopause, but the results from this study suggested the effects of testosterone at the point of estrus return in the uterus following the synchronization of estrus, and this seemed to maximize uterus damage. Moreover, we observed that the action of TIMPs varied depending on the type of hormone hypersecreted and the response was very low in the PMSG treated group. Unexpectedly, the expression of TIMPs in the testosterone treated group was high compared to other groups. A similar result was seen by Grzechocinska et al. (2018), who reported that TIMPs expression increased in the abnormal endometrium (Grzechocinska et al., 2018). Our study was based on the hypothesis that the hypersecretion of PMSG, P4, and testosterone is associated with MMPs and can play a direct role in mediating changes in the uterus. The results of this study showed that PMSG and testosterone highly increased the activity of MMPs, thereby influencing the physiological function of the uterus and affecting morphological changes in the uterus. Our study showed that the hypersecretion of hormones, which are important in reproductive physiology, plays a key role in the remodeling in the uterus. Although the exact genetic mechanisms were not elucidated in this study, we believe that hypersecretion of hormones may affect not only PCOS but also affect the uterus. These findings can be used to regulate endometrial remodeling.
Acknowledgment
This study was conducted materially in the Reproductive & Biotechnology Laboratory of the Hankyung National University in Korea.
Authors Contribution
SH Kim participated in developing the protocol, the sample granulosa cell, and in drafting the manuscript. SH Kim participated in the development of the database. SH Kim and JT Yoon contributed to the translation of the manuscript. JT Yoon supervised the analysis.
Conflict of interest
The authors declare that they have no conflict of interest.
References