Advances in Animal and Veterinary Sciences
Research Article
The Impacts of Partial Replacement of Sacha Inchi Seed on Growth Performance, Fatty Acids Composition, Blood Parameters, Histological Changes, and Immune-Related Gene Expression in Nile Tilapia (Oreochromis niloticus)
Buntheourn Khen1,2, Niran Aeksiri1,2, Kunlayaphat Wuthijaree1, Kumrop Ratanasut1,2, Gen Kaneko3, Anurak Khieokhajonkhet1,2*
1Department of Agricultural Science, Faculty of Agriculture, Natural Resources, and Environment, Naresuan University, Phitsanulok 65000, Thailand; 2Center of Excellence in Research for Agricultural Biotechnology, Naresuan University, Phitsanulok 65000, Thailand; 3School of Arts and Sciences, University of Houston-Victoria, 3007 N. Ben Wilson, Victoria, TX 77901, USA.
Abstract | The objective of this study was to determine the effects of partial replacement of fishmeal with sacha inchi seed (SIS) meal on growth performance, fatty acids composition, blood parameters, histological changes, and immune-related gene expression in Nile tilapia. Three iso-nitrogenous and iso-lipidic diets were formulated: a control diet without SIS replacement (SIS0) and two experimental diets with 29 (SIS29) and 54 (SIS54) g kg-1 of SIS replacement. A total of 180 fingerlings Nile tilapia with an average body weight of 11.10 ± 0.02 g/fish were randomly divided into 3 groups with 4 replicates at a density of 15 fish per replicate, and each group was fed one of the three diets to apparent satiation twice daily for 10 weeks. The results showed that dietary inclusion of SIS did not cause significant differences in growth and somatic indices (P > 0.05). However, the SIS29 diet significantly increased the protein efficiency ratio (PER) and apparent digestibility coefficient of dry matter (ADC of dry matter). There were no significant differences (P > 0.05) in hematocrit and red blood cell count, but hemoglobin and white blood cells (WBCs) were significantly increased by SIS replacement (P < 0.05). Dietary SIS inclusion also significantly decreased triglyceride (TAG) and low-density lipoprotein cholesterol (LDL-c) (P < 0.05). The hepatic expression of mRNAs encoding TNFα, Interleukin-1 (IL-1), and TGFα genes was not significantly affected (P > 0.05) by SIS inclusion, although the TNFα mRNA was slightly up-regulated. Whole-body fatty acid profiling demonstrated that the SIS54 group has significantly higher levels of C18:3n-3, 20:5n-3, and total n-3 than other groups. These results suggest that partial inclusion of SIS can improve the nutritional quality by increasing n-3 fatty acid contents without any negative effects on growth performance in Nile tilapia.
Keywords | Sacha inchi seed, Growth, Fatty acids, Gene expression, Nile tilapia
Received | July 22, 2021; Accepted | August 02, 2021; Published | December 01, 2021
*Correspondence | Anurak Khieokhajonkhet, Department of Agricultural Science, Faculty of Agriculture, Natural Resources, and Environment, Naresuan University, Phitsanulok 65000, Thailand; Email: anurakk@nu.ac.th
Citation | Khen B, Aeksiri N, Wuthijaree K, Ratanasut K, Kaneko G, Khieokhajonkhet A (2022). The impacts of partial replacement of sacha inchi seed on growth performance, fatty acids composition, blood parameters, histological changes, and immune-related gene expression in nile tilapia (oreochromis niloticus). Adv. Anim. Vet. Sci. 10(1): 94-106.
DOI | http://dx.doi.org/10.17582/journal.aavs/2022/10.1.94.106
ISSN (Online) | 2307-8316; ISSN (Print) | 2309-3331
Copyright © 2022 Khieokhajonkhet et al. This is an open access article distributed under the Creative Commons Attribution License, which permits unrestricted use, distribution, and reproduction in any medium, provided the original work is properly cited.
INTRODUCTION
In modern food science, functionality is considered an important criterion for food quality along with stability and palatability. This is also the case in fish breeding, which has drawn our attention to dietary ingredients that directly affect the growth and development of target species in aquaculture (Back et al., 2020). Fishmeal is one of such high-quality ingredients in fish feed and has been used as the main protein source in the livestock feed industry for many years (El-Sayed, 2020; Tacon, 1993). In addition to the extraordinary quality protein for aquatic animals, fishmeal also contains high levels of unsaturated fatty acids (DHA, docosahexaenoic acid and EPA, eicosapentaenoic acid) and essential amino acids such as lysine, which is often lacking in the grain products (Tantikitti et al., 2016; Zinn et al., 2009). Fishmeal also has high digestibility, palatability, and no anti-nutritional factors (Alexis and Nengas, 2001; NRC, 2011; Villanueva-Gutiérrez et al., 2020). The main benefits of fishmeal feeding include improving immunity, reducing incidences of deformities to animal health, and increasing survival and growth. However, along with the increased demand for fishmeal, the price has risen influencing not only the fish aquaculture but also the husbandry of crustacean and livestock production. Fishmeal is now one of the most expensive ingredients in commercial feeds (Amaya et al., 2007; Olsen et al., 2014).
Currently, natural ingredients are increasingly being used in aquatic feed to enhance immunity and fish health (Neamat-Allah et al., 2021; Mahmoud et al., 2020). Sacha inchi (Plukenetia volubilis) is an oleaginous plant from the tropical rainforest of the Amazon region, which is being commercially planted in South-East Asia, most remarkably in Thailand (Saengsorn and Jimtaisong, 2017). Sacha inchi seed (SIS) is reported to have the potential of being a cost-effective fish feed ingredient based on the nutritional values (Araujo-Dairiki et al., 2018); SIS has high oil (41.1%) and protein contents (24.7%) (Gutiérrez et al., 2011), and the oil is rich in omega-3 linolenic acid (45%–53%), omega-6 linoleic acid (34%–39%), and omega-9 (6%–10%) fatty acids (Wang et al., 2018). Furthermore, the ratio of ω-6 and ω-3 of the SIS is close to the ideal value (1 : 4 to 1 : 10), which exerts the hypo-cholesterolemic effect (Souza et al., 2019). Sacha inchi has been known as a beneficial food owing to the predominance of unsaturated fatty acids, and there are many attempts to use it as a feed ingredient, including those for human consumption.
Plant proteins, which are widely available and moderately priced, maybe the most realistic option in this regard. For the thriving global aquaculture sector, there is continuing interest in exploring and developing appropriate plant protein sources to the high feed cost of fishmeal (Khieokhajonkhet et al., 2021). However, the high inclusion of plant protein sources might cause an adverse effect on growth, feed utilization, and health status in fish due to imbalanced amino acid profiles and the presence of anti-nutritional factors (ANFs) (El-sayed, 1999; Adeoye et al., 2016). Previous studies reported that SIS pressed cake contains many ANFs, e.g. saponin, tannin, phytic acid, and trypsin inhibitor which usually have adverse effects on feed intake, growth performance, and feed and nutrient utilization (Rawdkuen et al., 2016; Khieokhajonkhet et al., 2021). Raw and air-dried SIS pressed cake thus could reduce growth performance and feed utilization, also causing histological changes of the intestine in Nile tilapia (Muichanta et al., 2020; Khieokhajonkhet et al., 2021). In contrast, Nile tilapia fed extruded SIS pressed cake could replace soybean meal in Nile tilapia (Khieokhajonkhet et al., 2021). Similarly, Ortiz-Chura et al., reported that rainbow trout fed extruded SIS pressed cake showed 98% apparent digestibility of protein (Ortiz-Chura et al., 2018). It is thus likely that although SIS contains a high concentration of ANFs, these can be removed or deactivated by appropriate treatments, making SIS a valuable alternative protein source for aquatic animals. However, there is only one report on SIS inclusion in fish diet, which used juvenile tambaqui (Colossoma macropomum) and matrinxã (Brycon amazonicus) (Araujo-Dairiki et al., 2018).
Nile tilapia (Oreochromis niloticus) is comprehensively cultured worldwide and recognized as one of the most important aquaculture resources due to the durability, incredible flexibility, capability to reproduce in a wide range of physical and environmental conditions, superb growth rates on a wide range of natural and prepared diets, resistance to handling and disease-causing agents, and their broad consumer appeal as a food fish (Khieokhajonkhet, 2020). The present study aims to determine the effects of substituting fishmeal with SIS on growth, fatty acids composition, blood parameters, histological changes, and immune-related genes expression in Nile tilapia.
MATERIALS AND METHODS
Experimental Diets
Air-dried SIS was purchased from the Ultimate Bankok Co.Ltd., Bangkok, Thailand. SIS was ground using a kitchen blender and kept at ‒20˚C until used. Three (isonitrogenous and isolipidic) diets were formulated as shown in Table 1. The control diet was formulated with equal contributions of fishmeal and soybean meal to the protein content. The two experimental diets were prepared by replacing 29 and 54 g kg-1 of fishmeal with SIS (corresponding to SIS29 and SIS54, Table 1). These numbers were determined based on the crude fat requirement of Nile tilapia. In order to meet the amino acids requirement of Nile tilapia, DL-methionine and L-lysine were supplemented to all experimental diets. All dry feed ingredients were thoroughly mixed using the C-B20G-A1 feed blender (Nonthaburi, Thailand). Soybean oil was added as the main energy source. After the addition of 350 ml kg‒1 distilled water, the resultant mixtures were subjected to a meat mincer to produce 3 mm diameter pellets using an ICK family meat mincer (Nonthaburi, Thailand). The pellets were air-dried, packed in plastic bags, and stored at ‒20°C. The proximate composition and fatty acid profiles of experimental diets are shown in Table 1 and Table 2, respectively. To determine the fatty acids composition in experimental diets and whole-body fish, total lipids were extracted in duplicate (n = 2) with chloroform-methanol (2 : 1, v/v) following Floch et al. (1957). Fatty acid methyl esters (FAME) were trans-esterified using 2% H2SO4 in methanol and then detected by gas chromatography using flame ionization detection (GC-FID, GC-2010 gas chromatograph, Shimadzu, Tokyo, Japan).
Experimental Fish
Nile tilapia (O. niloticus) fingerlings were purchased from a local hatchery farm (Dokdin Hatchery Farm, Phitsanulok, Thailand). Fish were transported and acclimatized under quarantine conditions in two 500-L fiber tanks for a month. The care and maintenance of experimental fish were conducted according to the Guide of the Care and Use of Laboratory protocol. The procedure was approved by The National Animal Care, Ethics, and Use Committee of Naresuan University, Phitsanulok, Thailand (protocol number: NU-AQ620202). For acclimatization, fingerlings were fed to apparent satiation with the commercial diet (containing approximately 30% protein and 4% lipid) for herbivorous fish (NB Distribution Co, Ltd., Ratchaburi, Thailand) twice daily at 09:00 and 16:00.
At the initiation of the feeding trial, all fish in each tank were weighed and randomly distributed into 100-L tanks (15 fish per tank, 4 replicates per treatment). During the feeding trial, fish were fed up to apparent satiation with experimental diets twice daily at 09:00 and 16:00. The feeding trial was continued for 10 weeks at the Aquatic Animal Feed Laboratory, Faculty of Agriculture, Natural Resources, and Environment, Naresuan University, Phitsanulok, Thailand. All tanks had continuous aeration, and the following water quality parameters of each tank were measured twice a week: temperature (23.27 ± 2.37ºC), dissolved oxygen (6.47 ± 1.35 mg/l), and pH (6.80 ± 0.59).
Sample Collection
After 10 weeks, fish were starved for 24 h and anesthetized with 50 mg/ml of clove oil (1 : 9 ratio of clove oil : ethanol). Fish were counted and weighed to calculate survival rate and growth parameters. After that, whole-body fish were air-dried and used for the determination of whole-body proximate composition following AOAC (1990). Four fish were randomly collected from each dietary treatment (pooled sample, n = 4), anesthetized, and used for hematological analysis. Blood samples were withdrawn from the individual aquaria at the caudal venipuncture using heparinized 1 ml syringes and immediately analyzed under using a light microscope (Olympus, Tokyo, Japan). For blood biochemistry, collected blood samples were stood for 15 min and centrifuged at 5000 rpm for 10 min. Blood serum was transferred in fresh tubes and stored at ‒20 for later analysis. Two fish were randomly collected from each of the four triplicate tanks (n = 8 per dietary treatment), weighed individually, and their liver and visceral organ were collected to determine organosomatic indexes. For gene expression analysis, liver tissue samples were collected from eight individual fish (2 fish of individual tank, n = 8) and stored in RNAlater (Ambion, Cambrideshier, UK) at ‒80˚C until further analysis. Anterior intestine samples were also fixed in 10% buffered formalin for histological analysis.
Analytical Methods
Growth Parameters and Somatic Indices: Growth performance, nutrient utilization, survival, and somatic indices of fish were calculated using the following equations: Weight gain (WG, %) = [final body weight (FBW, g) – initial body weight (IBW, g)] / IBW (g) × 100; Specific growth rate (SGR, % / day) = [ln (FBW, g) – ln (IBW, g) / number of days] × 100; Feed conversion ratio (FCR) = feed intake (g) / weight gain (g); Survival Rate (%) = [final number of surviving fish / initial number of fish] × 100; Protein efficiency ratio (PER) = weight gain / protein intake; Protein productive value (PPV, %) = protein gain (g) / protein intake (g); Condition factor (K, g/cm3) = individual FBW (g) / (individial body length)3 × 100; Hepatosomatic index (HSI, %) = individual liver weight (g) / individual body weight of fish (g) × 100; Viscerosomatic index (VSI, %) = individual viscera weight (g) / total individual fish weight (g).
Apparent Digestibility Analysis
The ADC of three experimental diets was examined by using chromic oxide (Cr2O3) as the indicator at a level of 5 g / kg diet. Fish were fed with diets containing Cr2O3 during week 8th - 10th of the feeding trial, and debris and uneaten feed were taken out. After being fed the diets containing Cr2O3 for 3 days, fresh feces were immediately collected by siphoning (Lin et al., 2004) and kept at ‒20˚C until used. The feces were dried at 60°C for 12 h and used for proximate analyses. Chromic oxide in diets and feces were determined by following the chromic oxide method in Furukawa and Tsukahara (1966). ADCs of nutrients were calculated using the following equations: ADC of dry matter (ADC of dry matter) = 100 ‒ (100 × % Cr2O3 content of diet / % Cr2O3 content of feces); ADC of protein = 100 – 100 × [(Cr2O3 content in diet) / (% crude protein content in diet)] × [(% crude protein content in feces) / (% Cr2O3 content in feces)].
Blood Analysis
Red blood cells (RBCs) and white blood cells (WBCs) were manually counted by diluting blood samples to 1000 and 100 times, respectively, with 0.85% NaCl, under a light
Table 1: Feed formulation and proximate composition of experimental diets.
Items | Experimental diets | |||
SIS0 | SIS29 | SIS54 | ||
Fish meal | 170 | 156 | 144 | |
Sacha inchi seed | 0 | 29 | 54 | |
Soybean meal | 370 | 370 | 370 | |
Corn gluten | 194 | 194 | 194 | |
Rice flour | 173 | 170 | 167 | |
Soybean oil | 58 | 46 | 36 | |
Vitamin premix1 |
10 | 10 | 10 | |
Mineral premix2 |
10 | 10 | 10 | |
Methionine | 10 | 10 | 10 | |
Lysine | 5 | 5 | 5 | |
Total | 1000 | 1000 | 1000 | |
Proximate composition (%) |
SIS3 |
SIS0 | SIS29 | SIS54 |
Dry matter | 98.00 ± 0.47 | 92.30 ± 0.59 | 92.09 ± 0.89 | 92.07 ± 0.62 |
Crude protein | 25.16 ± 0.66 | 29.51 ± 0.37 | 29.40 ± 0.99 | 29.43 ± 0.76 |
Crude lipid | 46.79 ± 0.83 | 6.98 ± 0.21 | 7.00 ± 0.36 | 7.69 ± 0.03 |
Crude ash | 2.82 ± 0.05 | 7.77 ± 0.10 | 7.55 ± 0.08 |
7.44 ± 0.23 |
1SunMix, Munkong Interfeed, Nakorn Pratom, Thailand (mg or IU/kg diet): A, 25,000 IU; D3, 1,500 IU; E, 1,000 mg; K, 50; B1, 30 mg; B2, 50 mg; B6, 15 mg; B12, 150 mg; inositol, 15 mg; pantothenic acid, 50 mg; niacin acid, 20 mg; C, 500 mg; folic acid, 5 mg; biotin, 3 mg.
2MinMin, Munking Interfeed, Nakorn Pratom, Thailand, Mineral premix (g/kg diet): calcium lactate, 1000; ferrous sulphate, 15; potassium chloride, 2.5; potassium iodine, 10; copper sulphate, 150; manganese oxide, 15; cobalt carbonate, 5; zinc oxide, 20, magnesium chloride, 26; sodium selenite, 15.
3SIS, raw material purchased from the Ultimate Bangkok Co. Ltd., Bangkok. Thailand.
Table 2: Fatty acids composition of experimental diets (g/kg fatty acids).
Fatty acids | SIS0 | SIS29 | SIS54 |
Saturated fatty acids (SFA) | |||
C6:0 | 0.2 | 0.2 | 3.0 |
C8:0 | 0.1 | 0.1 | 0.1 |
C10:0 | 0.1 | 0.2 | 0.2 |
C12:0 | 0.8 | 0.8 | 0.7 |
C14:0 | 4.8 | 4.3 | 4.0 |
C15:0 | 0.6 | 0.5 | 0.5 |
C16:0 | 126.5 | 122.3 | 110.4 |
C17:0 | 1.9 | 1.8 | 1.9 |
C18:0 | 67.3 | 66.5 | 65.1 |
C20:0 |
5.8 | 4.6 | 5.7 |
C22:0 | 4.7 | 0.0 | 3.3 |
C23:0 | 0.4 | 0.0 | 0.0 |
C24:0 | 2.0 | 2.1 | 1.2 |
Monounsaturated fatty acids (MUFA) |
|||
C16:1 | 0.6 | 0.5 | 0.4 |
C18:1n-9 cis | 25.0 | 23.8 | 21.6 |
C20:1n-9 | 0.4 | 0.4 | 0.4 |
Polyunsaturated fatty acids (PUFA) | |||
C18:2n-6 cis | 301.0 | 356.7 | 361.4 |
C20:2n-6 | 0.0 | 0.8 | 0.8 |
C18:3n-6 | 3.9 | 3.6 | 3.4 |
C18:3n-3 |
99.9 | 136.3 | 190.5 |
C20:4n-6 | 0.5 | 0.4 | 0.4 |
C20:5n-3 | 1.3 | 1.0 | 1.0 |
C22:5 | 0.6 | 0.4 | 0.4 |
C22:6n-3 |
3.0 | 2.5 | 2.1 |
∑SFA | 215.2 | 203.4 | 196.1 |
∑MUFA | 26.1 | 24.8 | 22.5 |
∑n-3 | 104.2 | 139.8 | 193.6 |
∑n-6 | 305.4 | 361.5 | 366.0 |
∑n-9 | 25.4 | 24.2 | 22.0 |
∑n-3/∑ n-6 |
0.34 | 0.38 |
0.53 |
Table 3: Nucleotide sequences of primers used in the present study.
Genes |
Primer sequences (5’→3’) |
Product size | References |
TNF-α |
F-GCTGGAGGCCAATAAAATCA R- CCTTCGTCAGTCTCCAGCTC |
339 bp | |
IL-1β |
F-AAGATGAATTGTGGAGCTGTGTT R-AAAAGCATCGACAGTATGTGAAAT |
175 bp | FF280564.1 |
TGF-β |
F- TGCGGCACCCAATCACACAAC R- GTTAGCATAGTAACCCGTTGGC |
105 bp | |
β-actin |
F-ACAGGATGCAGAAGGAGATCACAG R-GTACTCCTGCTTGCTGATCCACAT |
155 bp |
Table 4: Growth performance and biological indices of Nile tilapia fed experimental diets for 10 weeks.
Parameters | SIS0 | SIS29 | SIS54 |
P – value |
Growth and nutrients utilization | ||||
Initial body weight (g/fish) | 11.10 ± 0.02 | 11.11 ± 0.01 | 11.10 ± 0.02 | 0.601 |
Final body weight (g/fish) | 51.90 ± 3.23 | 54.47 ± 3.25 | 51.38 ± 1.10 | 0.278 |
Weight gain (%) |
367.50 ± 28.40 | 390.21 ± 29.09 | 363.00 ± 10.40 | 0.285 |
Specific growth rate (%/day) | 2.20 ± 0.09 | 2.27 ± 0.09 | 2.19 ± 0.03 | 0.281 |
Feed conversion ratio | 1.27 ± 0.17 | 1.24 ± 0.16 | 1.32 ± 0.04 | 0.712 |
Survival rate (%) | 100.00 ± 0.00 | 97.78 ± 3.85 | 95.56 ± 3.85 | 0.296 |
Protein efficiency ratio |
2.78 ± 0.02b |
3.05 ± 0.01a |
2.79 ± 0.01b |
< 0.000 |
Protein productive value (%) |
46.60 ± 1.05a |
48.58 ± 0.90a |
43.57 ± 1.67b |
0.001 |
Somatic indices | ||||
Condition factor (K, g/cm3) |
1.67 ± 0.07 | 1.77 ± 0.04 | 1.75 ± 0.11 | 0.955 |
Hepatosomatic index (%) | 1.46 ± 0.34 | 1.53 ± 0.11 | 1.58 ± 0.40 | 0.523 |
Viscerosomatic index (%) | 6.72 ± 1.81 | 6.66 ± 0.97 | 6.86 ± 0.85 | 0.949 |
Apparent digestibility coefficients (ADCs) | ||||
ADC of dry matter (%) |
73.03 ± 0.79b |
74.02 ± 0.08a |
71.64 ± 0.63c |
0.001 |
ADC of protein (%) |
90.38 ± 0.31a |
91.10 ± 0.35a |
88.47 ± 0.54b |
0.001 |
Data of growth and nutrient utilization, somatic indices, and ADCs are presented as mean value ± SEM (n = 4). The P – value column indicates P values from ANOVA. Means with different superscripts indicate significantly differences in Duncan’s test (P < 0.05).
Table 5: Whole-body composition of Nile tilapia fed experimental diets for 10 weeks (%, wet weight basis).
Composition | Initial fish | SIS0 | SIS29 | SIS54 |
P - value |
Dry matter |
23.23 ± 0.68 | 26.72 ± 0.42 | 26.85 ± 0.15 | 26.28 ± 0.39 | 0.386 |
Protein | 13.04 ± 0.49 |
15.81 ± 0.18a |
15.33 ± 0.24b |
15.06 ± 0.68b |
0.003 |
Fat | 3.43 ± 0.09 |
4.30 ± 0.33b |
4.77 ± 0.22a |
4.67 ± 0.43a |
0.004 |
Ash | 3.69 ± 0.24 |
3.51 ± 0.13a |
3.29 ± 0.26b |
3.46 ± 0.11a |
0.001 |
Data presented as mean value ± SEM (n = 4). The P – value column indicates P values from ANOVA. Means with different superscripts indicate significantly differences in Duncan’s test (P < 0.05).
Table 6: Whole body fatty acids composition of Nile tilapia fed experimental diets for 10 weeks (g/kg fatty acids).
Fatty acids | SIS0 | SIS29 | SIS54 |
P - value |
Saturated fatty acids (SFA) | ||||
C6:0 |
1.8b |
3.1a |
2.9a |
0.024 |
C8:0 |
0.5c |
0.7b |
0.9a |
< 0.001 |
C10:0 |
0.1b |
0.2a |
0.2a |
0.008 |
C12:0 |
4.1b |
4.9ab |
5.9a |
0.022 |
C13:0 |
0.0b |
0.1b |
0.2a |
0.006 |
C14:0 |
21.4a |
20.2b |
20.3ab |
0.041 |
C15:0 | 4.8 | 4.2 | 4.3 | 0.248 |
C16:0 | 137.5 | 133.3 | 126.7 | 0.067 |
C17:0 | 7.5 | 7.0 | 6.8 | 0.306 |
C18:0 | 81.3 | 83.9 | 79.7 | 0.432 |
C20:0 | 5.3 | 5.8 | 6.5 | 0.180 |
C22:0 |
0.0b |
2.6a |
2.7a |
0.009 |
C24:0 |
0.0b |
0.0b |
0.7a |
0.049 |
Monounsaturated fatty acids (MUFA) | ||||
C14:1 |
1.6b |
2.1b |
3.2a |
0.007 |
C16:1 | 30.1 | 26.4 | 27.4 | 0.075 |
C18:1n-9 cis |
248.8a |
238.1ab |
232.9b |
0.038 |
C20:1n-9 |
15.3a |
13.0b |
13.4b |
0.025 |
C24:1 |
0.0c |
0.6b |
0.9a |
< 0.001 |
Polyunsaturated fatty acids (PUFA) | ||||
C18:2n-6 cis |
238.8a |
220.9b |
210.1c |
< 0.001 |
C18:3n-6 | 12.4 | 15.5 | 15.8 | 0.116 |
C18:3n-3 |
38.1c |
50.5b |
74.6a |
0.002 |
C20:2n-6 | 15.4 | 13.9 | 13.5 | 0.072 |
C20:3n-3 | 5.4 | 8.1 | 16.4 | 0.131 |
C20:4n-6 |
14.8a |
11.5b |
9.2b |
0.007 |
C20:5n-3 |
0.0b |
1.1a |
1.2a |
0.004 |
C22:5 | 4.2 | 3.8 | 3.5 | 0.397 |
C22:6n-3 | 11.4 | 12.0 | 12.5 | 0.272 |
∑SFA | 264.3 | 266.0 | 257.9 | 0.051 |
∑MUFA |
295.9a |
280.3b |
278.0b |
0.008 |
∑n-3 |
55.0c |
71.8b |
104.8a |
0.002 |
∑n-6 |
281.4a |
261.8b |
248.6c |
< 0.001 |
∑n-9 |
264.1a |
251.1b |
246.4b |
0.022 |
∑n-3/∑ n-6 |
0.19a |
0.27b |
0.42c |
0.001 |
Data presented as mean value ± SEM (n = 2). The P – value column indicates P values from ANOVA. Means with different superscripts indicate significantly differences in Duncan’s test (P < 0.05). Abbreviation: SIS, sacha inchi seed; SFA, saturated fatty acids, MUFA, monounsaturated fatty acids; PUFA, polyunsaturated fatty acids, ∑SFA, summation of saturated fatty acids; ∑MUFA, summation of monounsaturated fatty acids; ∑n-3, summation of n-3 fatty acids; ∑n-6, summation of n-6 fatty acids; ∑n-9, summation of n-9 fatty acids.
Table 7: Hematological and serum biochemical parameters of fish fed experimental diets for 10 weeks.
Parameters | SIS0 | SIS29 | SIS54 |
P - value |
Hematological parameters | ||||
Hemoglobin (mg/dL) |
4.62 ± 0.16c |
5.23 ± 0.40b |
5.85 ± 0.05a |
< 0.001 |
Hematocrit (%) | 34.27 ± 3.41 | 36.53 ± 2.58 | 37.81 ± 0.87 | 0.187 |
RBC (106 cell/mm3) |
1.39 ± 3.27 | 1.31 ± 3.05 | 1.51 ± 2.30 | 0.478 |
WBC (103 cell/mm3) |
119.44 ± 1.92b |
123.44 ± 7.99b |
150.62 ± 1.95a |
< 0.001 |
Blood biochemistry | ||||
Glucose (mg/dL) | 83.50 ± 0.58 | 84.50 ± 6.35 | 71.00 ± 19.63 | 0.635 |
Total protein (g/dL) | 3.22 ± 0.26 | 2.88 ± 0.04 | 2.77 ± 0.20 | 0.280 |
Albumin (g/dL) | 1.34 ± 0.08 | 1.19 ± 0.06 | 1.15 ± 0.07 | 0.216 |
Globulin (g/dL) | 1.88 ± 0.18 | 1.68 ± 0.04 | 1.62 ± 0.11 | 0.052 |
AST (U/L) | 35.21 ± 2.38 | 35.42 ± 0.98 | 32.11 ± 0.62 | 0.286 |
ALT (U/L) | 20.93 ± 0.16 | 19.76 ± 0.71 | 17.27 ± 1.27 | 0.081 |
Cholesterol (mg/dL) | 153.71 ± 2.96 | 150.66 ± 5.18 | 151.33 ± 1.68 | 0.782 |
TAG (mg/dL) |
104.29 ± 4.79a |
75.50 ± 0.58b |
88.56 ± 4.06b |
0.017 |
HDL-c (mg/dL) | 71.71 ± 1.69 | 76.09 ± 3.07 | 71.31 ± 4.54 | 0.508 |
LDL-c (mg/dL) | 81.85 ± 8.46 | 60.46 ± 3.37 | 62.66 ± 5.21 |
0.110 |
Values are mean value ± SEM (n = 4). The P – value column indicates P values from ANOVA. Means with different superscripts indicate significantly differences in Duncan’s test (P < 0.05). Abbreviations: SIS, sacha inchi seed; RBC, red blood cell; WBC, white blood cell; AST, aspartate aminotransferase; ALT, alanine aminotransferase; TAG, triglycerol; HDL-c, high-density lipoprotein cholesterol; LDL-c, low-density lipoprotein cholesterol.
Table 8: Intestinal morphometric measurements of Nile tilapia fed experimental diets for 10 weeks.
Parameters | SIS0 | SIS29 | SIS54 |
P - value |
Villus height (μm) |
360.71 ± 11.96 | 345.95 ± 19.61 | 357.35 ± 12.87 | 0.501 |
Villus width (μm) |
109.86 ± 5.00b |
155.24 ± 34.51a |
105.90 ± 12.17b |
0.044 |
Serosa (μm) |
53.37 ± 0.82b |
59.70 ± 3.45a |
44.67 ± 1.58c |
0.001 |
Longitudinal muscle layer (μm) |
34.07 ± 0.68 | 36.07 ± 2.30 | 36.28 ± 3.31 | 0.494 |
Values are mean ± SEM (n = 8). The P – value column indicates P values from ANOVA. Means with different superscripts indicate significantly differences in Duncan’s test (P < 0.05).
microscope using Neubauer Hematocytometer (Drabkin, 1945). Hematocrit was performed using a glass capillary tube with one end sealed by centrifuging samples at 2600 × g for 5 min with a DM1424 microhematocrit centrifuge (DLAB, Beijing, China). Hemoglobin levels were determined according to the colorimetric method (Blaxhall and Daisley, 1973). Blood biochemical parameters were determined according to the colorimetric method using blood chemical analyzer kits (Stanbio Laboratory, Boerne, TX, USA) and a blood serum analyzer (P400 and PC400, Horiba, Japan) as described by Coz‐Rakovac et al. (2008).
Histological Examination
Fixed tissue samples were processed using the standard histological practices. Briefly, samples were dehydrated in ethanol, infiltrated in xylene, and embedded in paraffin wax. The tissue blocks were sectioned serially using a rotary microtome. Then, the sectioned tissues were stained with hematoxylin and eosin (H&E). The stained slides were then observed using a light microscope (Olympus, Tokyo, Japan). To evaluate the intestinal integrity, quantitative image analysis was conducted by following a previous study (Martínez-Llorens et al., 2012) using the AxioVision software (Carl Zeiss, Jena, Germany). The villus height, villi width, serosa thickness, and the longitudinal muscle layer were measured.
Rna Extraction And Real-Time Quantitative Polymerase Chain Reaction (Qrt-Pcr)
Frozen tissues samples, approximately 2-3 g, were homogenized in liquid nitrogen using pestle and mortar. The fine powder was immediately dissolved in 1 mL Qiazol Lysis Reagent (Qiagen, Maryland, USA). Total RNAs were extracted using RNeasy Mini Kit by following the manufacturer’s protocol (Qiagen, Hilden, Germany). The concentration and purity of obtained total RNAs were spectrophotometrically assessed using a microplate reader (Synergy H1 Multi-Mode Reader, VT, USA). RNA integrity was visualized by agarose gel electrophoresis. Total RNAs were then reverse-transcribed to cDNA using a RevertAid First-Strand Synthesis System (Thermo Scientific, Fermentas, USA) according to the manufacture’s protocol. Quantitative real-time PCR was carried out to determine the relative mRNA levels of TNFα, IL-1β, and TGF-α in the liver of Nile tilapia fed different diets. The gene-specific primers of TNFα, IL-1β, TGF-α, and β-actin genes are shown in Table 3. β-actin was used as an internal control gene, and its amplification efficiency was ensured based on a cycle threshold value (Ct-value) using serial dilution (R2 > 0.99). The real-time PCR was performed according to the manufacturer’s recommendation of Maxima SYBR Green/ROX qPCR Master Mix (2X) (Thermo Fisher Scientific, Vilnius, Lithuania). The reaction was performed in 20 µl reaction mixtures containing 3 µl of diluted cDNA template (1 : 100 dilution with sterile distilled water), 10 µl of Maxima SYBR Green/ROX qPCR Master Mix (2X) (Thermo Fisher Scientific, Vilnius, Lithuania), 10 pmol of gene-specific primers, and 5 µl of sterile distilled water. The PCR cycling profiles were as follows: enzyme activation was carried out at 95°C for 3 min, followed by 40 cycles of denaturing at 95°C for 3 s and extension at 60°C for 30 s. The absence of non-specific PCR products was ensured by the dissociation curve. The relative mRNA expression of fish in different treatments was determined by the comparative Ct-value method.
Statistical Analysis
All data were shown as mean ± SEM. One-way ANOVA was used for testing significant differences. Duncan’s multiple range test was used to identify which means values were significantly different from each other. Differences were considered significant at P < 0.05. All data were statistically analyzed using SPSS (Chicago, IL, USA) for Window version 17.0.
RESULTS
Growth Performance And Biological Indices
Dietary replacement of fishmeal by SIS had no significant effects on growth performance (P > 0.05, Table 4). In addition, there were no significant differences (P > 0.05) in survival rates among all treatments. The fish fed the SIS29 diet showed higher PER and PPV than those fed other diets (P < 0.05), while the SIS54 group showed the lowest PPV. Similarly, no gross pathological changes of visceral organs were observed in all experimental fish. Also, no statistical differences were observed for any of the K, HSI, or VSI among treatments. Fish fed the SIS29 diet had significantly higher ADC of dry matter and ADC of protein than those of the other groups (P = 0.001).
Whole-Body Composition
The whole-body compositions of Nile tilapia fed with the three different levels of SIS in diets are presented in Table 5. After 10 weeks of the feeding trial, the moisture content showed no significant differences among all treatments (P > 0.05). The whole-body protein content of fish fed the control diet was significantly higher compared to fish fed SIS-containing diets (P = 0.001). Fat contents were significantly increased in the SIS diet groups compared to the control group (P = 0.004), while ash content was low in the SIS29 diet group (P = 0.001).
The fatty acids composition of Nile tilapia after the 10-week trial is shown in Table 6. Saturated fatty acids (SFA) levels were not significantly different among all treatments (P > 0.05). MUFA was significantly decreased with the increasing amount of SIS in the diets (P = 0.008). Moreover, n‐3 PUFA was significantly increased by the replacement of fishmeal with SIS (P = 0.002), leading to a concomitant increase significantly in an n‐3/n‐6 ratio (P = 0.001). Conversely, n-6 and n-9 PUFA were significantly decreased (P < 0.001, P = 0.022) in Nile tilapia fed with the SIS-containing diets compared to those fed the control diet (Table 6).
Hematological and Serum Biochemical Parameters
Hematological and serum biochemical parameters are presented in Table 7. Nile tilapia fed the control diet (SIS0) showed a significantly lower hemoglobin concentration and WBCs (P < 0.001) compared to fish fed the SIS29 or SIS54 diets. There were no significant differences (P > 0.05) in hematocrit and RBC values as well as most blood serum parameters (glucose, total protein, albumin, globulin, cholesterol, HDL-c, and LDL-c) except for the triglycerol (TAG) values. The TAG value was significantly lower in SIS groups compared to the control group (P = 0.017). There were no significant differences in AST and ALT activities (P > 0.05).
Intestinal Histology and Morphology
Histological images of the intestine of Nile tilapia fed SIS inclusion in diets are shown in Figure 1. Dietary SIS inclusion groups showed microvilli with increased size and
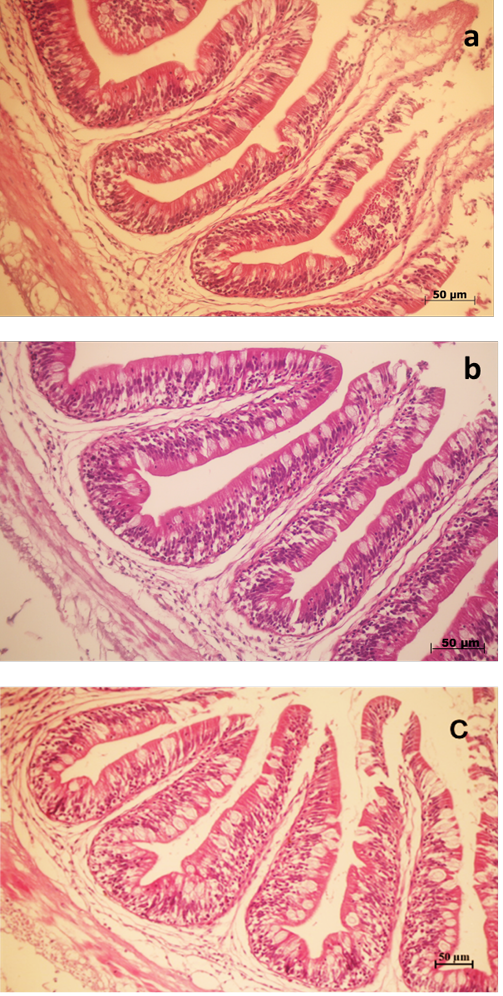
Figure 1: Stained intestinal tissue section (H&E) of Nile tilapia fed three different level of sacha inchi seed in diets for 10 weeks. (a) intestinal tissue of Nile tilapia fed SIS0 diet (b) intestinal tissue of Nile tilapia fed SIS29 diet (c) intestinal tissue of Nile tilapia fed SIS54 diet.
number of goblet cells (Figure 1B, 1C). Intestinal morphometrics showed no significant changes in intestinal villus height among the three groups (P < 0.05, Table 8). Nile tilapia fed the SIS29 diet showed significantly higher villus width and serosa layer than the other two groups (P = 0.044, 0.001). There were no significant differences among all treatments in the longitudinal muscle layer (P > 0.05).
Gene Expression
The expression of genes related to immunity (TNF-α, IL-1β, and TGF-β) was assessed by real-time PCR in Nile tilapia after the feeding trial (Figure 2). The expression of TNF-α was slightly increased in SIS inclusion groups but the differences were not significant (P > 0.05). The expression of IL-1β and TGF-β genes also showed no significant difference between groups (P > 0.05).
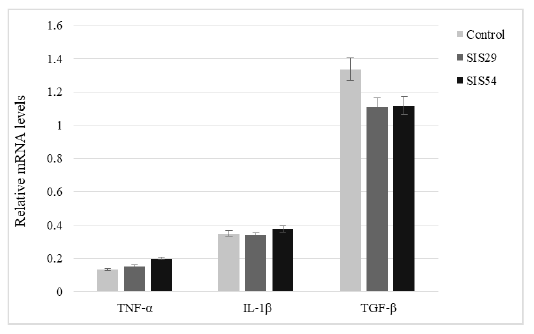
Figure 2: Relative expression of TNF-α, IL-1β, and TGF-β of Nile tilapia fed experimental diets for 10 weeks.
DISCUSSION
The present study determined the effects of fishmeal substitution by SIS at different inclusion levels of 0, 29, and 54 g kg‒1 in Nile tilapia diets. The results revealed that fishmeal replacement with SIS up to 54 g kg‒1 did not cause any significant differences in growth parameters, although Nile tilapia fed the SIS29 diet showed the highest growth. This is not very surprising because the inclusion of sacha inchi at 150 and 300 g kg-1 did not cause any significant changes in growth parameters in Tamaqua and Matrinxã (Araujo-Dairiki et al., 2018). This is probably because Nile tilapia has a high requirement for n-6 fatty acids compared to n-3 fatty acids for optimal growth (Ochang et al., 2007). The SIS supplementation increased dietary n-3 but not n-6 fatty acid levels in whole-body fish, and thus were expected to have minor effects on growth. Survival rates of fish fed SIS-based diets showed no significant differences compared to fish fed the control diet, suggesting that dietary SIS supplementation does not influence fish mortality. For future applications, it is also important to identify the upper limit of SIS inclusion in fish diets.
In fish, the condition factor is used as a valuable index for monitoring various factors including feeding intensity, age, and growth rates (Oni et al., 1983). Hepatosomatic index (HSI) and viscerosomatic index (VSI) are used to determine the health status of fish (Sogbesan et al., 2017). HSI is influenced by several factors such as water quality, nutrition, stocking density, and stress (Panase et al., 2018). In this present study, these biological indices did not show significant differences among all treatments. These responses suggest that inclusions of SIS in the diet up to 54 g kg‒1 did not have major adverse effects. On the other hand, the apparent digestibility coefficient of dry matter and ADC of protein of fish fed SIS29 were significantly higher than those of control and SIS54 groups. Ortiz-Chura et al. (2018) reported that sacha inchi is the most acceptable ingredient for juvenile trout according to the ADC values of the protein and the digestible energy compared to other potential feed ingredients.
Replacement of fish meal or fish oil with non-conventional ingredients also commonly affects fatty acids composition (Gbadamosi and Lupatsch, 2018; Takeuchi et al., 2002; Youssouf et al., 2011). In the present study, dietary inclusion of SIS54 resulted in significantly higher 20:5n-3, n-3 fatty acids, and n-3/n-6 ratio (P < 0.05) compared to other groups. These results could be linked to higher amounts of 18:2n-6 and 18:3n-3 in diets which is a precursor to synthesize n-3 LC-PUFAs, such as eicosapentaenoic acid (20:5n-3, EPA), docosapentaenoic acid (22:5n-3, DPA), and docosahexaenoic acid (22:6n-3, DHA) (Chen et al., 2018; Petenuci et al., 2018), through desaturation and elongation processes (Francis et al., 2007). In addition, it has been documented that freshwater fish such as Nile tilapia and catfish can manufacture the longer chain n-3 HUFA, EPA, and DHA from 18:2n-6 and 18:3n-3, which are necessary for other metabolic functions and as cellular membrane components (Craig et al., 2017; Tocher, 2010). This is in an agreement with the previous study in which n-3 LC-PUFAs and Σ n-3/n-6 PUFA increased significantly when fish oil was replaced by sacha inchi oil (Lima et al., 2019).
This present investigation of blood parameters demonstrated that the WBC values of fish fed SIS up to 54 g kg-1 is significantly increased compared to fish fed the control diet. The amount of WBCs is a disease marker, which is related to inflammatory stimulus and immune system (Liang et al., 2018). At the same time, several previous reports showed that increased WBC counts could be a protective response to stress (Das, 1998) and a consequence of improved immune response (Gabriel et al., 2009). It is not possible to determine whether the observed increase in WBC value indicates the disease state or improved immune response, but the concomitant increase in the expression levels of immune-related genes suggests that substituting fishmeal with inclusion levels up to 54 g kg-1 of SIS can be a consequence of improved immune response.
AST and ALT enzymes are usually localized inside cells of various organs and tissues such as the liver, heart, gills, kidneys, muscle, and other organs (Hadi et al., 2009). When cells in these organs, typically hepatic cells, are damaged, a huge amount of ALT and AST is released into the bloodstream. Thus, serum levels of ALT and AST can be used as an indicator of liver cell damage (Soltan et al., 2008). Moreover, the AST and ALT levels are demonstrative of general systemic nutritional status, vascular system integrity, and liver function (Kumar et al., 2011). In the present study, no significant changes in the AST and ALT activity were observed in fish fed the SIS-containing diets compared to fish fed the control diet. These results indicate that SIS inclusion in diet did not damage liver cells, which is consistent with no significant differences in cholesterol, HDL-c, and LDL-c levels. On the other hand, TAG levels were significantly decreased. This is possibly related to the increased n-3 fatty acid contents because long-chain n-3 fatty acids are known to reduce plasma TAG levels (Shearer et al., 2012).
The present study demonstrated that dietary SIS inclusion at 29 g kg-1 could significantly increase the number of goblet cells compared with those of other diets. Previous studies mentioned that a high number of goblet cells could increase the ability to nutrient absorption and intestinal healthiness (Peng et al., 2013; Ismail et al., 2020). In addition, dietary inclusions of SIS did not cause significant differences in villus height and longitudinal muscle layer, but significant differences were detected in villus height and serosa space. The increase in the intestinal villi length could improve the absorptive surface area, resulting in better nutrient utilization of O. niloticus (El-latif et al., 2015). Indeed, increased villus length has been associated with improved tilapia growth performance (Elsabagh et al., 2018). In this study, the increase in growth observed in the SIS29 group did not reach statistical significance during the 10-week experimental period. However, the changes in intestinal morphology may exert some positive effects on growth in the long term, which should be tested in future studies.
As a pro-inflammatory cytokine, TNF-α plays an important role in cell proliferation, differentiation, necrosis, apoptosis, and the induction of other cytokines (Rahman and McFadden, 2006). It is used as a marker of innate immune system function and tissue damage. IL-1β is the best-categorized cytokine amongst the 11 mammalian IL-1 family members. It is considered as one of the earliest expressed pro-inflammatory cytokines and plays a central role in the initiation of systemic and local responses to infection or injury by activating macrophages, T and B lymphocytes, and NK cells (Dinarello, 2011; Netea et al., 2010). These function of IL-1β overlaps with the functions of TNFα, enabling the organism to respond promptly to infection (Zou and Secombes, 2016). Transforming growth factor-β (TGF-β) is a pleiotropic polypeptide that regulates cell development, migration, adult stem cell differentiation, immune regulation, wound healing, and inflammation (Kastin, 2013; Reyes-Cerpa et al., 2013). In this study, the expression level of these genes (TNF-α, IL-1β, and TGF-β) was not significantly influenced by dietary treatments, indicating that substituting fishmeal with SIS up to 54 g kg‒1 in Nile tilapia diet did not affect the immune response. Future research is also needed to focus on the effects of various levels of SIS in Nile tilapia diets until we see adverse effects.
CONCLUSION
In conclusion, the results exhibited that SIS levels up to 54 g kg-1 (approximately 15% of fishmeal) did not affect growth performance, somatic indices, intestinal morphology, and gene expression of target genes of Nile tilapia. However, substituting fishmeal with SIS improved fatty acid profiles by increasing the amount of HUFAs in fish muscle. These results suggest that SIS could be an alternative feed ingredient by improving nutritional quality for aquaculture.
ACKNOWLEDGEMENTS
This research was supported by Royal Scholarship under Her Royal Highness Princess Maha Chakri Sirindhorn Education Project to the Kingdom of Cambodia.
CONFLICT OF INTEREST
All authors declare that there are no conflicts of interest.
AUTHORS CONTRIBUTION
Methodology, Investigator, B.T., A.K.; Blood analysis, N.A.; Writing-Review, Funding Acquisition, Supervision, Conceptualization, A.K.; Editing, Final approve, B.T., K.W., K.R., G.K., A.K.; Proofreading, G.K.; All authors have read and approved the final version of the article.
REFERENCES