Advances in Animal and Veterinary Sciences
Review Article
Assessing and Mitigating the Impact of Heat Stress in Poultry
Sachin Shivaji Pawar1, Sajjanar Basavaraj1, Lonkar Vijaysinh Dhansing2, Kurade Nitin Pandurang1*, Kadam Avinash Sahebrao2, Nirmale Avinash Vitthal1, Brahmane Manoj Pandit1, Bal Santanu Kumar1
1ICAR-National Institute of Abiotic Stress Management, Malegaon, Baramati 413115, Maharashtra, India; 2Department of Poultry Science, K.N.P. College of Veterinary Science, Shirwal 412801, Maharashtra, India.
Abstract | Over the years, development of poultry genotypes is driven mainly aiming higher production at optimal temperatures. However, recent increase in extreme heat wave events and enhanced sensitivity of modern poultry genotypes to heat stress has become a major concern leading to significant economic losses to the poultry industry. In India, per capita consumption of poultry meat and eggs is significantly lower than the minimum recommended by Indian Council of the Medical Research and there is need for significant increase in poultry productivity to fulfil these requirements. Conversely, in sub-tropical countries like India where summer is severe, accomplishing higher production under current scenario is a challenge. Earlier intervention strategies including environmental management and nutritional supplementation have been inconsistent in poultry for managing heat stress. Therefore, there is scope for exploring innovative approaches, including genetic marker-assisted selection of poultry breeds for increased heat tolerance and application of molecular techniques in poultry breeding to improve poultry productivity in a sustainable manner. Hence, keeping in view the present situation, it is essential to understand various cellular and molecular mechanisms involved in poultry production including physiological and immunological aspects of the poultry birds under heat stress using molecular biology tools that can help in development of poultry breeds which are better adapted to changing climate.
Keywords | Poultry, Heat stress, Mitigation
Editor | Kuldeep Dhama, Indian Veterinary Research Institute, Uttar Pradesh, India.
Received | May 03, 2016; Accepted | June 13, 2016; Published | June 17, 2016
*Correspondence | Kurade Nitin P, ICAR-National Institute of Abiotic Stress Management, Malegaon, Baramati 413115, Maharashtra, India; Email: npkurade@yahoo.co.in
Citation | Pawar SS, Sajjanar B, Lonkar VD, Nitin KP, Kadam AS, Nirmale AV, Brahmane MP, Bal SK (2016). Assessing and mitigating the impact of heat stress in poultry. Adv. Anim. Vet. Sci. 4(6): 332-341.
DOI | Http://dx.doi.org/10.14737/journal.aavs/2016/4.6.332.341
ISSN (Online) | 2307-8316; ISSN (Print) | 2309-3331
Copyright © 2016 Pawar et al. This is an open access article distributed under the Creative Commons Attribution License, which permits unrestricted use, distribution, and reproduction in any medium, provided the original work is properly cited.
Domestication of poultry is believed to have begun in Asia where poultry was kept by farmers in India, China and East Asia long before it was known to Europeans and Americans (VanWulfetenPalthe, 1992). Chickens have been bred in captivity in Egypt since 1400 BC. The earliest record of poultry in India dates back to 3200 BC and it is believed that today’s chicken breeds have originated in India (Daghir, 2008). These breeds have supposedly arisen from a common ancestor, the red jungle fowl which is found in the wild in the forests of India and South-East Asia (West and Zhou, 1989). Today, poultry farming has become an important activity throughout the world. As per the Food and Agriculture Organisation (FAO), the annual global poultry meat production was estimated to be 103.5 million tonnes in 2012 which is 34.3% share of global meat production (excluding fish). The poultry meat and egg production system is considered to be the most efficient animal protein production system with the smallest carbon foot print per unit product produced. Poultry industry has for some time occupied a leading role among agricultural industries in many parts of the world (Daghir, 2009). In the recent times, poultry industry is being viewed as a provider of a healthy alternative to red meat and other protein sources (Williams et al., 2006).
In the wake of rising consumption of poultry products and its widespread production, stressors including abiotic factors can have negative impacts on the productivity and health of chickens thereby affecting nutrition of humans. Due to the steadily rising global average surface temperature with expected rise of 1.4 to 5.8°C by 2100 Today, heat stress has emerged as the major concerns in poultry industry since (IPCC, 2007) and concentration of poultry industry in hot climate areas of Asia and South America (Daghir, 2008). Over the years, long term single-trait selection for yields has resulted in modern poultry genotypes with greater metabolic activity, producing more body heat and lower heat tolerance. The performance of the modern birds, especially broilers is vastly different from the broiler of three decades ago wherein, the modern day broiler grows more than three times faster than the vintage broiler (Havenstein et al., 1994). Modern poultry genotypes developed over the years produce more body heat due to their superior metabolic activity which makes them more sensitive to temperature related environmental changes (Settar et al., 1999; Deeb and Cahaner, 2002). Under circumstances such as high heat, high humidity with reduced air flow or any other extreme stress, the death rate due to physiological changes has increased (Julian 1993). The birds when exposed to heat stress leads to alteration in behavioural, physiological and immunological responses which inflict unfavourable effect on their overall productivity, which is more pronounced during hot seasons (Mazzi et al., 2003; Lara and Rostagno, 2013). The losses due to heat stress in broilers include high mortality, diminished growth and egg production, deterioration in meat and egg quality and safety. The awareness and concern regarding negative impact of heat stress on poultry welfare has enhanced gradually in recent times (Lara and Rostagno, 2013). Therefore, understanding effects of heat stress on poultry productivity, developing effective mitigation strategies to reduce resulting production losses, and deciphering the genetic basis of thermos-tolerance aiming development of heat resilient poultry breeds has become crucial for successful poultry production and welfare especially in sub-tropical climatic regions.
Poultry Farming Scenario in India
Though the country has achieved food grain security for entire population, an estimated 24 million undernourished people are yet to solve the problem of poor nutrition especially protein and mineral associated health issues in lower income groups (Lupien and Menza, 1999). Poultry meat being the cheapest source of proteins with few social taboos associated with poultry meat and eggs consumption, poultry sector has potential to provide food and livelihood securities to major chunk of Indian population (Paswan et al., 2014). The development of poultry sector in the country has been more rapid than that of any other animal sector with more than 10% rate of increase (Daghir, 2009) and contributes nearly 45% of the total production of meat in the country (Figure 1).
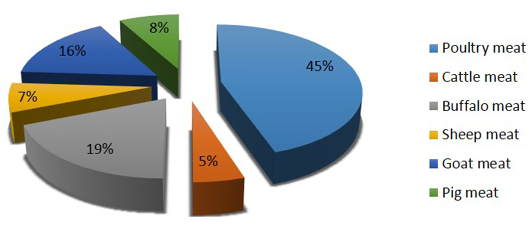
Figure 1: Species wise meat contribution in India (Source: Basic animal husbandry & fisheries statistics, 2014)
Poultry development has shown a steady progress over the last four decades and has transformed from a mere backyard activity into a major commercial activity with state-of-the-art technological interventions (DAHD&F, 2013). This transformation has involved significant investments in breeding, hatching, rearing and processing of poultry products. Farmers in India have moved from rearing non-descript birds to rearing hybrids which ensure faster growth, good liveability, better feed conversion and high profits (Mehta et al., 2002). Presently, India has emerged as the 3rd largest producer of egg and 5th largest producer of poultry meat in the world as compared to 30th and 12th positions, respectively in 1980s. Presently, Indian poultry industry is valued about 35 thousand crore rupees (BAHS, 2010; Prabakaran, 2012). With increasing demand for poultry, this sector employs around 1.6 million people. At least 80% of employment in the poultry sector is generated directly by these farmers, while 20% is engaged in feed, pharmaceuticals, equipment and other services. Additionally, there may be a similar number of people roughly 1.6 million who are engaged in marketing and other channels servicing this sector (Mehta et al., 2002). About 70% of the poultry production, management and marketing in India are under a highly organized sector (Statistical Year Book, India 2014). The number of poultry birds has increased from 307 million in 1992 to 729.2 million in 2012 with increase of 12.39% from 2007 (Figure 2).
During the 1990s and early 2000s, production and consumption of poultry products in India increased by about 11% as compared to a global growth rate of 5% (Landes, 2002). In 2011-12, the production of poultry egg and meat in the country was around 66.45 billion and 2.47 million tonnes, respectively. Poultry sector also contributes significantly to the country’s export and as per the report of Agricultural and Processed Food Products Export Development Authority (APEDA), exports of poultry products in 2011-12 were estimated at around 457.82 crore rupees.
Mechanisms of Body Heat Regu-lation in Poultry
The metabolic activities involved in maintenance, growth and egg production in birds leads to heat production and is influenced by species, breed, body weight, level of production, level of feed intake, feed quality and, to a lesser extent, by the amount of activity and exercise (DEFRA, 2005). The regulation of body heat in birds mainly occurs through five mechanisms namely, radiation, conduction, convection, evaporation and excretion (DEFRA, 2005; Halls, 2014). Heat lost through radiation is by electromagnetic means wherein, if the surrounding temperature is below bird surface temperature heat will radiate from the bird’s warmer body to a cooler surface, such as air, without the use of a medium. Conversely, hot walls and roofs may radiate heat to the bird surfaces (Mustaf et al., 2009). During hot weather, birds raise their wings to allow heat to radiate from poorly feathered areas, such as under the wings. Body heat loss through convection i.e., by moving air over birds is the most effective way to reduce heat stress as the natural rise of warm air from around the bird’s hot body will cause heat loss. Providing moving air can assist convection, but only if the air moves fast enough to break down the boundary layer of still air that surrounds the body (Lara and Rostagno, 2013). Proper ventilation is the key to keeping birds cool in hot weather. Heat loss through evaporation is very important at high temperatures as poultry do not sweat but depend on panting for losing heat. Water evaporation occurs on the surface of the skin and from the respiratory tract. The nasal cavity is a heat exchanger which helps rid the body of excess heat through evaporative cooling; hence panting is regarded as the most obvious clinical sign of heat stress in poultry (Fedde, 1998). However, panting is only effective if the humidity is not too high. Therefore, hot, humid conditions are much more stressful than the hot dry conditions. Excretion is another method used by birds to lose body heat wherein, birds normally double their water intake during hot periods and excrete heat through urine and wet faeces (DEFRA, 2005; Halls, 2014).
Due to the rise in ambient temperature, a wide variety of physiological, behavioural, neuroendocrine and molecular responses are initiated to maintain body temperature within the normal limits. In some instances, the responses are short-term measures that are invoked to withstand a brief period of extreme temperature. The responses can also be invoked to develop a longer-term response aimed at acclimatizing the bird to ambient temperatures that fall within the upper regions of the thermoregulatory zone. Lastly, the terminal response comes in play that can be only short-lived and initiation of these responses helps to cope with environmental variables which are extreme and lethal (Daghir, 2008).
Heat Stress in Poultry
Stress is defined as the “nonspecific response of the body to any demand”, whereas stressor can be defined as “an agent that produces stress at any time” (Selye, 1976). Hence, stress represents a biological response of the animal/organism to stimuli that disturb its normal physiological equilibrium or homeostasis. The optimum temperature for performance/ thermoneutral zone is between 19-22ºC for laying hens and 18-22ºC for growing broilers (Charles, 2002). Poultry birds when are in ‘thermoneutral zone’, do not suffer from heat stress as body temperature is held constant and the birds lose heat at a controlled rate using normal behaviour. However, any deviation from the thermoneutral zone results in heat stress, causing a negative balance between net amount of energy flowing from the bird’s body to its surrounding environment and the amount of heat energy produced by the bird (Figure 3).
Heat stress can occur at all ages and in all types of poultry breeds. Birds must lose heat actively when the upper critical temperature is exceeded however, if heat production becomes greater than ‘maximum heat loss’ either in intensity (acute heat stress) or over long periods (chronic heat stress), it can be fatal to birds (DEFRA, 2005; Lara and Rostagno, 2013). Heat stress is caused by variations of a combination of environmental factors such as, sunlight, thermal irradiation, air temperature, humidity; characteristics of the bird e.g., breed, metabolism rate, bird’s activity and thermoregulatory mechanisms as well as the housing conditions of bird (Lara and Rostagno, 2013). The various signs indicating heat stress in poultry includes, gasping, panting, spreading of wings, lethargic and droopy acting, extremely pale cones and wattles, closed eyes, lying down, drop in egg production, reduced egg size, egg weight, poor shell quality, increased thirst, decreased appetite, loss of body weight and increased cannibalism (Dayyani and Bakhtiari, 2013).
Impact of Heat Stress on Production
Due to higher production performance and feed conversion efficiency, today’s chickens are more susceptible to heat stress than ever before (Lin et al., 2006). In addition, the tropical areas of Asia and South America have become centre of poultry industry presently (Daghir, 2008). The physiological, behavioural and immunological responses generated when the birds are exposed to high environmental temperatures significantly affect their productivity. Heat stress causes reduced feed intake, activation of hypothalamic-pituitary-adrenal (HPA) axis and modification of neuroendocrine profile of bird, thereby affecting overall poultry and egg production. Individual variation in intensity and duration of responses may be noticed in birds based on intensity and duration of the heat stress, however; birds react similarly to heat stress in general (Lara and Rostagno, 2013). Several studies have documented poor growth performance in broilers subjected to heat stress (Deeb and Cahaner, 2002; Attia et al., 2011; Ghazi et al., 2012). Chronic heat stress in broilers causes significant reduction in feed intake, lowered body weight and higher feed conversion ratio (Sohail et al., 2012). The deterioration of meat quality traits due to heat stress occurs mainly in consequence of the associated higher rate of lipid peroxidation and the altered electrolyte balance (Babinszky et al., 2011). In layers, the detrimental effects of heat stress are intiated with decreased feed intake followed by reduced dietary digestibility, and decreased plasma protein and calcium levels, causing significant damage to egg production and quality. (Mahmoud et al., 1996; Zhou et al., 1998; Mashaly et al., 2004; Lin et al., 2006). Heat stress has been demonstrated to decrease production performance in layers causing reduced eggshell thickness, egg shell thickness, increased egg breakage and egg weight and percentage (Ebeid et al., 2012).
Along with production losses, reproductive performance of the poultry is affected in several ways in response to heat stress. In females, reduced systemic levels of reproductive hormones and their impaired functions are demonstrated resulting from disruption of normal status of these hormones at the hypothalamus and ovary (Donoghue et al., 1989; Novero et al., 1991). In males, decreased semen volume, sperm concentration, number of live sperm cells and motility is reported in response to heat stress resulting in impaired fertility (Joshi et al., 1980; McDaniel et al., 1995). Although, the figures for overall losses to the Indian poultry industry due to heat stress are unavailable, it is reported that U.S. poultry industry loses estimated $128 to $165 million as a result of heat stress (St-Pierre et al., 2003) highlighting the economic impact of heat stress on poultry production.
Effect of Heat Stress on Immune Response
When bird’s body temperatures are in thermos-neutral zone, the energy from the feed can be directed to immune system development apart from the growth and reproduction (Dghir, 2008). During the heat stress, bird’s body makes several physiological changes to maintain body temperature causing reduced immune response (Daghir, 2008). In recent times, researchers have undertaken studies to investigate the effect of heat stress on immune response in poultry. Central nervous system (CNS) modulates the immune response by complex bi-directional networks operating between nervous, endocrine and immune systems. The immune response is mainly altered through pathways constituting the hypothalamic-pituitary-adrenal and sympathetic–adrenal medullar (SAM) axes (Lara and Rostagno, 2013). Overall, the studies show immunosuppressive effect of heat stress on broilers and laying hens e.g., reduced relative weights of thymus and spleen has been reported in laying hens and broilers subjected to heat stress (Bartlett and Smith, 2003; Quinteiro-Filho et al., 2010; Ghazi et al., 2012). Lower liver weights in laying hens (Felver-Gant et al., 2012) and lower levels of total circulating antibodies including specific IgM and IgG levels, both during primary and secondary humoral responses in broilers in response to heat stress is reported (Bartlett and Smith, 2003). Aengwanich (2008) reported reduced bursa weight along with decreased lymphocytes numbers in cortex and medulla of bursa in broilers subjected to heat stress. However, there is still inadequate understanding of immune response to heat stress in poultry with reference to genetic and cellular mechanisms.
Effect of Heat Stress on Food Safety
Food safety has become an important part in the modern day concept of food quality and more recently, the poultry industry worldwide is facing the major issue of food safety due to heat stress. Several evidences suggest that stress can have harmful effect on food safety through various potential mechanisms. There are several reports linking pathogen carriage and shedding in farms animals to stress, though the underlying mechanism is not fully revealed (Lara and Rostagno, 2013). The deleterious effect of heat stress in broilers by virtue of the associated undesirable meat characteristics and quality loss was reported earlier (Sandercock et al., 2001; Zhang et al., 2012). The meat quality losses were also reported during the transportation of broilers under high environmental temperature from farms to processing facilities (Mulder 1995; Dadgar et al., 2010). The negatively effect on egg production and quality in laying hens due to heat stress has been demonstrated earlier (Mashaly et al., 2004; Bozkurt et al., 2010).
Mitigation Strategies to Ameliorate Heat Stress in Poultry
Microclimate Modification: One of the keys to minimizing heat stress in poultry houses during hot weather is making sure that outside air can easily flow into and out of the house. The easier it is for outside air to flow through a house, the less likely there will be a detrimental build-up of heat within the house, minimizing inside to outside temperature differentials (Daghir, 2008). In hot and humid environments, open-style houses with proper shading, adequate air movement and water consumption are essential. Ventilation should be maximized as the air movement facilitates removal of build-up ammonia, carbon dioxide and moisture from the poultry sheds (Butcher and Miles, 2012). A grass cover on the grounds surrounding the poultry house will reduce the reflection of sunlight into the house. Vegetation should be kept trimmed to avoid blocking air movement and to help reduce rodent problems. Shade trees should be located where they do not restrict air movement. Another factor that affects heat gain of a house is the condition of the roof. A shiny surface can reflect twice as much solar radiation as a rusty or dark metal roof. Roofs should be kept free of dust and rust. Roof reflectivity can be increased by cleaning and painting the surface with metallic zinc paint or by installing an aluminium roof (Anderson and Carte, 2007). The use of circulation fans is recommended for proper ventilation. The primary purpose of circulation fans in a naturally ventilated house is not to bring air into the house but rather to produce air movement over the birds to increase convective cooling. Generally, it is best if circulation fans are orientated to blow with the long axis of a house and positioned towards the centre of the house, where air movement tends to be most needed. Circulation fans should generally be installed in rows. To maximize air movement over the birds, circulation fans should generally be installed 1–1.5 m above the floor and tilted downward at approximately a 5° angle (Daghir, 2008).
In poultry, not only the heat production but also heat loss is related to heat stress. Both, body heat production as well as body heat loss could be affected by management. Adequate ventilation is vital for heat stress management in poultry birds. Heat loss by evaporative heat dissipation is linked to relative humidity of the surrounding environment (Lara and Rostagno, 2013). Therefore, high temperature accompanied by high humidity is more detrimental to broiler performance than high temperature with low humidity. The evaporative heat loss increases along with temperature and decreases with increasing humidity (Lin et al., 2006). Early heat conditioning (EHC) seems to be one of the promising methods in enhancing heat resistance of broiler chickens. Early heat conditioning refers to the practice exposing broiler chicks to high temperature (36°C) for 24 h at 3 to 5 d of age (Lin et al., 2006). Where possible, and in particular in older broiler houses with less efficient ventilation, it is sound practice to reduce stocking densities in the summer (DEFRA, 2005).
Nutritional Supplementation: Feed conversion in broilers is subject to significant fluctuations because of seasonal as well as ambient temperature changes. All studies indicate that high temperature reduce the efficiency of utilizing feed energy for productive purposes. However, only part of the reduced performance of broilers is due to reduced feed intake and the rest is due to high temperature per se (Daghir, 2009). It is reported that the reduction in broiler growth due to reduced feed intake ranges from 63-67% (Dale and Fuller, 1979; Daghir, 2009). It is common practice now in formulating broiler feeds for hot regions to boost the energy level of these diets by adding fat. This practice not only increases the energy intake but also reduces the specific dynamic effect of the diet, which helps birds to cope better with heat stress (Daghir, 2009). Nutritional manipulations, such as addition of fat (Ghazalah et al., 2008) and reduction of excess protein (Rahman et al., 2002) are recommended to reduce the adverse effects of heat stress. The addition of fat to the diet also appears to increase the energy value of other feed constituents (Mateos and Sell, 1981) and has been shown to decrease the rate of food passage in the GI tract and thus increase nutrient utilization (Mateos et al., 1982). During hot periods, lower protein diets supplemented with limiting amino acids gives better results than the high protein diets. The supplementation of essential amino acids to a diet with poor quality protein or unbalanced amino acid profile helps performance by reducing heat increment and the harmful effects of high temperature. The poultry industry therefore has followed the practice of adjusting the dietary levels of protein and amino acids in order to assure an adequate intake of these essential nutrients (Daghir, 2009). Responses to amino acid supplements differ with different dietary factors at high temperature; dietary electrolytes being one of them (Chen et al., 2005).
Heat stress increases the mineral excretion from body and decreases the serum and liver concentrations of vitamins (e.g., vitamin C, E and A) and minerals (e.g., Fe, Zn, Se and Cr). Moreover, mobilization of minerals and vitamins from tissues and their excretion are increased under heat stress causing marginal mineral and vitamin deficiency (Sahin et al., 2009). Vitamins and mineral supplementation has been demonstrated to decrease mortality and improve growth of poultry birds during heat stress. The dietary electrolyte balance is more critical at high temperature than at normal temperature. Maintenance of both carbon dioxide and blood pH is vital to heat-stressed birds and the addition of ammonium chloride and potassium chloride to drinking water is desired to maintain this balance. The occurrence of alkalosis in heat stressed birds has been known for a long time and the addition of ammonium chloride, potassium chloride and/or sodium bicarbonate have improved performance of broilers by improving water and feed intake (Ahmad et al., 2008). The supplementation of NaHCO3, relieves the bicarbonate (HCO3–) deficiency brought about by a developing respiratory alkalosis resulted from panting (Benton et al., 1998). The addition of extra vitamins, electrolytes and antioxidants to the drinking water is also helpful during heat stress. Since heat stress always depresses appetite and therefore reduces nutrient intake, the use of a vitamin and electrolyte pack in the drinking water for 3-5 days during a heat wave has been shown to be helpful in most cases. Vitamin C (ascorbic acid) supplementation is probably the most beneficial among vitamins, and use of Vitamin C in the feed or in the drinking water has become a common practice in hot regions. Several nutritionists recommend the administration of 1g ascorbic acid/litre drinking water throughout heat periods. (Cier et al., 1992; Daghir, 2009).The detrimental effect of heat stress on egg production can also be alleviated by dietary supplementation of Vitamin A (8000 IU/kg diet) (Lin et al., 2002). Vitamin E supplementation is beneficial to the egg production of hens at high temperatures and is associated with an increase in feed intake and yolk and albumen solids (Kirunda et al., 2001). Heat stress could induce the unfavourable changes in indigenous bacterial microbiota (Lin et al., 2006). The supplementation of probiotic Lactobacillus strains may aid in restoring the gut microbial balance of chicken having suffered heat stress (Lan et al., 2004).
Apart from the nutrient supplementation, feeding practices (Moreki, 2008) suggested below are reported to improve performance of birds under heat stress. The good physical quality of feed (crumb, pellets or mash) is reported to encourage appetite. If there is enough floor space, extra feeders should be added. Feed should not be stored for longer than two months, especially in summer to reduce the possibility of mycotoxin build up. Practice feeding at cooler times of the day, i.e., early morning or in the evening. Feeding birds at cool times enables birds to make up for what they have not eaten during the day. Laying hens increase their calcium intake during the evening as eggshells are normally formed during this time. Remove feed 4 to 6 hours prior to an anticipated heat stress period. Birds should not be fed or disturbed during the hottest part of the day. Diming of lights while feeding or using low light intensity during periodical feeding reduces activity that reduces heat load.
Genetic Strategies: In the long term, single-trait selection for yields has resulted in lowered heat tolerance (Hoffmann, 2010). With increasing growth rates in poultry, metabolic heat production has increased and the capacity to tolerate elevated temperatures has declined (Zumbach et al., 2008; Dikmen and Hansen, 2009). Commercial production of chickens based on intensive selection for increased production efficiency has resulted in crowded production environments, thereby increasing bird’s exposure to heat stress (Cheng et al., 2004). In some cases, selection for production traits has increased the line’s susceptibility to stressors (Berrong and Washburn, 1998; DeSmit et al., 2005). Under the heat stress conditions, disease resistance of birds is often weakened due to the reduction in immunity (Shini et al., 2010).
There are several genes that affect heat tolerance. Some, such as the dominant gene for naked neck (Na), affect the trait directly by reducing feather cover (Cahaner et al., 1992), while others, such as the sex-linked recessive gene for dwarfism (dw), reduce body size and thereby reduce metabolic heat output. The frizzle (F) gene causes the contour feathers to curve outward away from the body. Deeb et al. (1993) reported that the F gene (as Ff) reduced the feather weight of broilers in addition to the reduction caused by the Na gene. The slow feathering (K) gene has been widely used to ‘auto-sex’ strain and breed crosses has effect on increased heat loss during early growth, all of which may assist the bird in resisting heat stress (Merat, 1990). At genetic level, when birds are exposed to thermal stress, the synthesis of most proteins is retarded, but a group of highly conserved proteins known as heat shock proteins (HSPs) is rapidly synthesized. In a heat-shocked cell, the HSP may bind to heat-sensitive proteins and protect them from degradation, or may prevent damaged proteins from immediately precipitating and permanently affecting cell viability (Etches et al., 1995). Polymorphism of heat shock protein genes based on SNP markers have been reported to be associated with heat tolerance of poultry birds. Identification of SNP markers can aid in marker assisted selective breeding of poultry birds for heat resilience (Yu et al., 2008; Chen et al., 2013).
For maintaining increasing trend of poultry production, improved thermos-tolerance along with disease resistance is always desired. The previous studied have revealed that genetic variations forms much of the basis for birds response to heat stress; however, knowledge in this area is limited and it needs further studies to explore its full potential (Felver-Gant et al., 2012; Lara and Rostagno, 2013). Genetic marker assisted selective breeding using the genes related to thermos-tolerance and disease resistance in poultry is the need of the hour for further enhancement in the poultry productivity.
Conclusions and Future Pros-pects
Although, the per capita consumption per annum of poultry, meat and eggs in India has shown a steady progress over the years and is presently estimated around 2.21 kg meat and 58 eggs, respectively (Table 1), is much lower than the minimum recommendations of 11 kg meat and 180 eggs/year person by Indian Council of the Medical Research (USDA, 2011). Attaining this mark needs raising poultry productivity by at least three times which still remains a major challenge in tropical country like ours where summer is severe. With the rising global temperature and lower heat tolerance of modern poultry genotypes, heat stress has emerged as a major concern causing significant economic losses to the poultry industry. Till date, various studies published in relation to heat stress in poultry, mainly focus on exploring the strategies to intervene conditions causing heat stress that include environmental management and nutritional supplementation. However, their effectiveness has been variable or uneven due to variability in age, breed, sex, management and geographical location.
Table 1: Production and availability of poultry meat and eggs in India during 2008-2013 (Source: Basic animal husbandry & fisheries statistics, 2014)
Year |
Production |
Per Capita Availability |
||
Meat (000 Tonnes) |
Eggs (Mil) |
Meat (kg / annum) |
Eggs (No / annum) |
|
2008-09 |
1884.41 |
55562 |
1.03 |
48 |
2009-10 |
2087.35 |
60267 |
1.78 |
51 |
2010-11 |
2193.14 |
63024 |
1.85 |
53 |
2011-12 |
2483.10 |
66450 |
2.05 |
55 |
2012-13 |
2681.60 |
69731 |
2.21 |
58 |
In recent times, newer methods are also being explored, including genetic marker assisted selection of poultry breeds for increased heat tolerance and disease resistance. Application of molecular techniques in poultry breeding has great potential to improve poultry productivity in a sustainable manner. Modern day molecular biology tools can help in understanding various cellular and molecular mechanisms involved in production, physiological and immunological aspects of the poultry birds, which in turn can help in development of breeds better adapted to changing climate. However, potential opportunities towards identification of new genetic markers along with the development of screening techniques for their identification still requires further research and development for successful and widespread applications aiming poultry development in hot climatic regions.
Acknowledgments
The authors sincerely thank Indian Council of Agricultural Research (ICAR), New Delhi, India and Director, ICAR-National Institute of Abiotic Stress Management, Baramati, India for providing necessary support for this work.
conflict of interests
The authors declare that there is no conflict of interest.
authors’ contribution
All authors contributed equally to the manuscript.
References