Advances in Animal and Veterinary Sciences
Research Article
Developmental Neurotoxicity of Acrylamide: Defensive Role of Chick Embryo Glutathione S-transferases
Sreenivasulu Dasari*, Muni Swamy Ganjayi, Balaji Meriga, Thyagaraju Kedam
Department of Biochemistry, Sri Venkatewara University, Tirupati, Andhra Pradesh, India.
Abstract | Acrylamide is a well proven neurotoxin and is a major environmental and food contaminant. Generally, birds are highly mobile in all environments and their developing embryo are the first victim of polluted environment caused by industrialization and agriculture system at various stages. However, data is lacking on birds species related to antioxidant defence system. The present study was aimed to analyze the potentiality of developing chick embryo’s brain glutathione S-tranferases (GSTs) to detoxify the toxic chemical agents such as acrylamide (AC). Findings of this study indicate that the high level expression of brain GSTs may detoxify the toxic chemical agents and therefore protect the brain. Excessive accumulation of toxic chemical agents such as AC can finally make injury to brain. Taken together, results of the study showed that as minimum as 6 parts per million of AC can cause cerebellar layers degeneration and vacuolation within 24 hours and 48 hours. Thus a minute quantity of AC is a potent neurotoxin for developing chicken embryos and efforts should be made to prevent such exposures for sufficient productivity of the poultry.
Keywords | Chick embryo, Brain GSTs, Acrylamide, Neurotoxicity, Brain injury
Editor | Kuldeep Dhama, Indian Veterinary Research Institute, Uttar Pradesh, India.
Received | May 07, 2017; Accepted | July 09, 2017; Published | July 25, 2017
*Correspondence | Sri Venkatewara University, Tirupati, Andhra Pradesh, India; Email: dasarisreenivaasulu@gmail.com
Citation | Dasari S, Ganjayi MS, Meriga B, Kedam T (2017). Developmental neurotoxicity of acrylamide: defensive role of chick embryo glutathione s-transferases . Adv. Anim. Vet. Sci. 5(7): 299-306.
DOI | http://dx.doi.org/10.17582/journal.aavs/2017/5.7.299.306
ISSN (Online) | 2307-8316; ISSN (Print) | 2309-3331
Copyright © 2017 Dasari et al. This is an open access article distributed under the Creative Commons Attribution License, which permits unrestricted use, distribution, and reproduction in any medium, provided the original work is properly cited.
Introduction
Overproduction of reactive oxygen species (ROS) and reactive nitrogen species (RNS) by either endogenous or exogenous agents is harmful to living beings (Valko et al., 2006). Cells are handling the ROS using glutathione antioxidant enzymatic system as it protect cells from oxidative stress (Limon-Pacheco et al., 2010; Ballatori et al., 2009). In bird species, detoxification efficiency is sometimes associated with the type of diet (Fossi et al., 1995). The xenobiotics cause noxious effects by direct interaction with specific sites on cellular macromolecules (e.g., enzymes) and interaction is dictated by the chemical nature of the toxicants (Liebler 2008; LoPachin and DeCaprio 2005). Acrylamide (AC) is a well proven neurotoxic chemical, for both central nervous system (CNS) and peripheral nervous system (PNS) (Sickles et al 2002). Both oxidative stress and apoptosis play a key role in AC induced toxicity (Sumizawa and Igisu, 2009; Alturfan et al., 2012; Lakshmi et al., 2012). The antioxidant ability is declined in both nervous tissue and sciatic nerve because of AC induced lipid peroxidation (Zhu et al., 2008). AC also induces apoptosis in cerebral cortex (Lakshmi et al., 2012). According to previous literature, AC is a carcinogen in addition to neurotoxicity and reproductive toxicity (Pourentezari et al., 2014; Sen et al., 2015).
Glutathione S-transferases (GSTs) (E.C.2.5.1.18) related phase II biotransformation enzymes which involved in cellular detoxification as well as excretion of various xenobiotic molecules (Eaton and Bammler, 1999; Frova, 2006); it is one of the key enzymes of glutathione antioxidant enzymatic system. Usually carcinogens, environmental toxins and also oxidative stress products are detoxified by GSTs which catalyse the conjugation of an electrophilic canter with reduced glutathione (GSH) (Konishi et al., 2005).
Cytosolic GSTs are dimeric proteins with 199–244 amino acids length and 23–30 kDa of molecular weight (Hayes and Pulford, 1995). Based on amino acid similarities mammalian cytosolic GSTs were classified as alpha (α), mu (μ), pi (π), theta (τ), sigma (σ), zeta (ζ) and omega (ο) (Frova, 2006;
Table 1: Brief procedure of model substrates specific activity with GST
Substrate |
Contents of reaction mixture |
Initiator |
Molar Extinction coefficient (cm-1) (Absorbance) |
CDNB |
1 ml of 0.3 M phosphate buffer (pH 6.5), 30 mM CDNB 100 μl, Enzyme 100 μl Total Vol 3ml |
30mM GSH 100μl |
9.6x103 (340 λ) |
BSP |
125 mM , phosphate buffer (pH 6.5), 0.003 mM BSP 100 μl, Enzyme: 200µg, Total vol 3 ml |
5 mM GSH 100μl |
4.5x1 03 (330 λ) |
pNBC |
1ml100 mM phosphate buffer (pH 6.5), 1mM pNBC 100 μl, Enzyme 200 μg, Total Vol. 3ml |
5 mM GSH 100 μl |
1.9x103 (310 λ) |
pNPA |
125 mM phosphate buffer (pH 7.0), 0.3 mM pNPA 100 μl, Enzme: 200 µg Total Vol. 3 ml |
0.5 mM GSH 100μl |
8.79x103 (400 λ) |
EPNP |
125 mM phosphate buffer (pH 6.5), 1mM EPNP 100 μl, Enzyme: 200 µg Total Vol. 3ml |
5 mM GSH 100 μl |
0.5x103 (360 λ) |
CHP |
1.8 ml assay buffer (50 mM Na2PO4 (pH 7), 2.5 mM EDTA, 2.5 mM NADPH), 5 mM GSH 100 μl & 100 μl GR, Enzyme 250 µg Total Vol. 3 ml |
1.2 mM 100μl CHP |
6.32x103 (340 λ) |
Table 2: Developing chick embryo GSTs protective role against AC induced neurotoxicity by 24 hours interval.
Model substrates |
Control |
2 ppm |
4 ppm |
6 ppm |
CDNB |
0.531± 0.021 |
0.704a± 0.041 |
0.852a± 0.032 |
0.203a± 0.026 |
BSP |
0.326± 0.023 |
0.482a ± 0.035 |
0.597a ± 0.046 |
0.107a ± 0.032 |
pNBC |
0.249 ± 0.021 |
0.386a ± 0.026 |
0.492a ± 0.031 |
0.103a ± 0.014 |
EPNP |
0.226 ± 0.024 |
0.383a ± 0.032 |
0.502a ± 0.032 |
0.146a ± 0.024 |
PNPA |
0.452 ± 0.042 |
0.696a ± 0.032 |
0.893a ± 0.053 |
0.297a ± 0.027 |
CHP |
0.321 ± 0.022 |
0.464a ± 0.034 |
0.597a ± 0.045 |
0.092a ± 0.023 |
The values which represented by table are average of three separate experiments of three samples. Mean ± standard deviation (SD). Student test (a = p<0.05 is regarded as significant specific activity of GST). AC was administered by doubling the dose 2 ppm per day by 24 hours interval.
Table 3: Developing chick embryo GSTs protective role against AC induced neurotoxicity by 48 hours interval.
Model substrates |
Control |
2 ppm |
4 ppm |
6 ppm |
CDNB |
0.597 ± 0.025 |
0.734a± 0.022 |
0.993a ± 0.033 |
0.202a ± 0.022 |
BSP |
0.278 ± 0.024 |
0.386a ± 0.026 |
0.597a ± 0.023 |
0.248a ± 0.028 |
pNBC |
0.206 ± 0.021 |
0.385a ± 0.025 |
0.496a ± 0.024 |
0.139a ± 0.023 |
EPNP |
0.248 ± 0.024 |
0.393a ± 0.021 |
0.487 a ± 0.019 |
0.136 a ± 0.026 |
PNPA |
0.398 ± 0.015 |
0.5973a ±0.022 |
0.783a ± 0.034 |
0.209a ± 0.025 |
CHP |
0.276± 0.023 |
0.394a ± 0.024 |
0.613 a ± 0.023 |
0.099a ± 0.026 |
The values which represented by table are average of three separate experiments of three samples. Mean ± standard deviation (SD). Student test (a = p<0.05 is regarded as significant specific activity of GST). AC was administered by doubling the dose 2 ppm per day by 48 hours interval.
Hayes et al., 2005). The avian GSTs possess a complex isoenzyme system, but researchers put little attention on them (Yeung and Gidari, 1980). Affinity purified chicken liver alpha class GSTs were partially cloned and characterized (Hsieh et al., 1999).
There are no considerable studies on antioxidant enzymes as well as indicators of oxidative stress in polluted environments in various bird species (Norte et al., 2009, Hegseth et al., 2011). Usually Birds have peculiar molecular mechanisms such as low rates of mitochondrial oxygen radical production and high blood glucose levels when compared to other vertebrates. Because of theses peculiar features birds can effectively defend free radicals and oxidative stresses (Pamplona and Costantini, 2011). The present aim is to study the acrylamide-induced developmental neurotoxicity and protective efficiency of chick embryo glutathione S-tranferases and degeneration of brain tissue.
Results
Acrylamide (Ac) Versus Developing Brain Glutathione S-Transferases (Gsts)
As shown in Table 2 and Figure 2 GSTs expressed against AC by 24 hours interval, shows specific activity with model substrates as follows. With CDNB, GSTs activities were significantly increased to 1.8 fold and 2.4 fold with 2 ppm and 4 ppm of AC, respectively and decreased to 3.2 fold with 6 ppm of AC than 5.2 fold of control. With BSP, GST specific activity was significantly increased to 1.6 fold and 1.8 fold with 2 ppm and 4 ppm of AC, respectively and decreased to 2.2 fold with 6 ppm of AC than 3.2 fold of control. With pNBC, GST specific activity was significantly increased to 1.2 fold and 2.3 fold with 2 ppm and 4 ppm of AC, respectively and decreased to 1.2 fold with 6 ppm of AC than 2.2 fold of control. With EPNP, GST specific activity was significantly increased to 1.3 fold and 2.4 fold with 2 ppm and 4 ppm of AC, respectively and decreased to 0.8 fold with 6 ppm of AC than 2.2 fold of control. With pNPA, GST specific activity was significantly increased to 2.6 fold and 4.6 fold with 2 ppm and 4 ppm of AC, respectively and decreased to 1.4 fold with 6 ppm of AC than 4.4 fold of control. With CHP, GST specific activity was significantly increased to 1.4 and 2.8 fold with 2 ppm and 4 ppm of AC, respectively and decreased to 2.0 fold than 3.2 fold of control.

Figure 1: Schematic diagram of glutathione S-transferases (GSTs) expression against acrylamide (AC) and tissue injury by AC
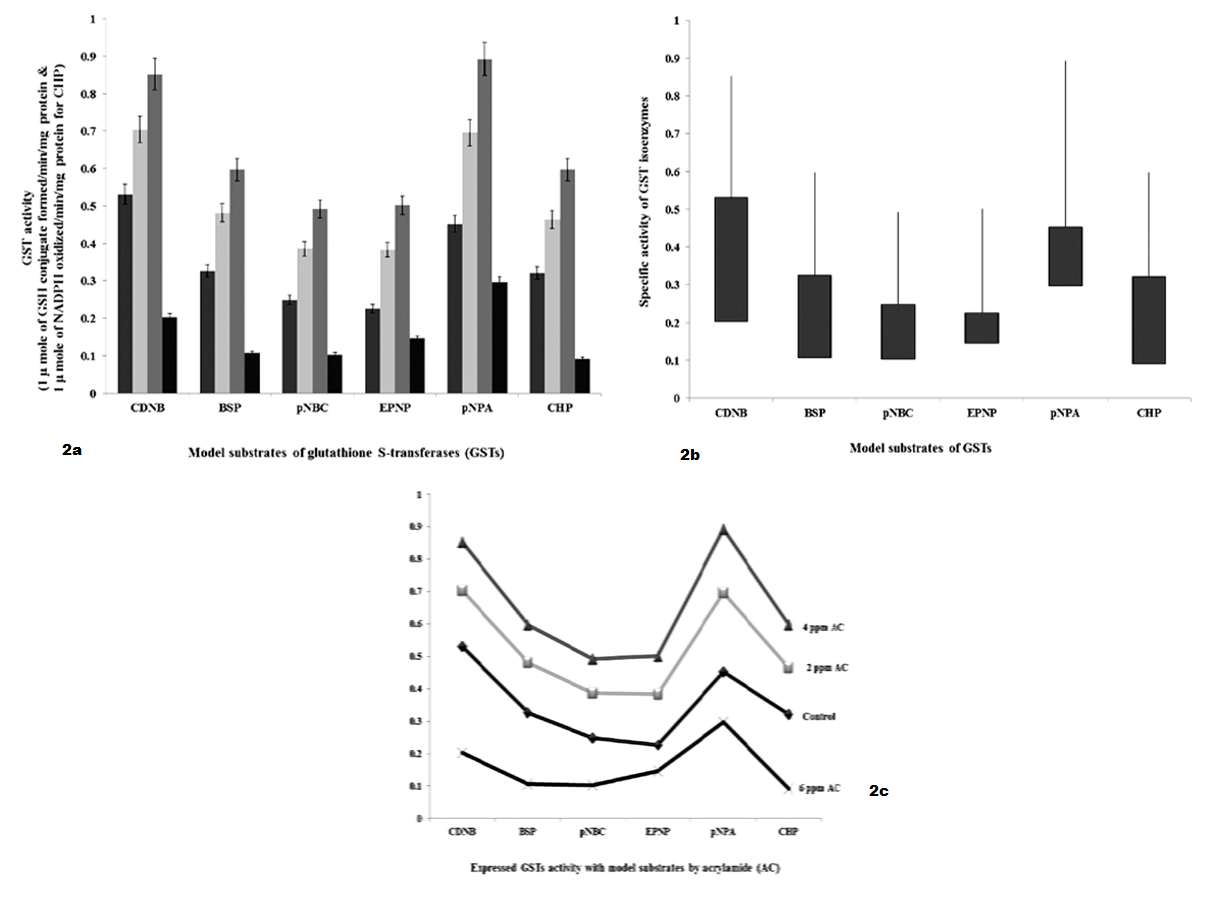
Figure 2 a): GSTs specific activity with model substrates by 24 hours intervening period; b): Differences in GSTs specific activity with model substrates by 24 hours intervening period; c): Expression levels of GST when administered with AC by 24 hours intervening period
As shown in Table 3 and Figure 3 GSTs expressed against AC by 48 hours interval shows, specific activity with model substrates as follows. With CDNB, GSTs activities were significantly increased to 1.34 fold and 4.0 fold with 2 ppm and 4 ppm of AC, respectively and decreased to 4.0 fold with 6 ppm of AC than 6.0 fold of control. With BSP, GST specific activity was significantly increased to 1.08 fold and 3.22 fold with 2 ppm and 4 ppm of AC, respectively and decreased to 0.3 fold with 6 ppm of AC than 2.78 fold of control. With pNBC, GST specific activity was significantly increased to 1.85 fold and 5.97 fold with 2 ppm and 4 ppm of AC, respectively and decreased to 0.6 fold with 6 ppm of AC than 2.0 fold of control. With EPNP, GST specific activity was significantly increased to 1.45 fold and 2.39 fold with 2 ppm and 4 ppm of AC, respectively and decreased to 1.12 fold with 6 ppm of AC than 2.48 fold of control. With pNPA, GST specific activity was significantly increased to 2.0 fold and 3.7 fold with 2 ppm and 4 ppm of AC, respectively and decreased to 2.0 fold with 6 ppm of AC than 4.0 fold of control. With CHP, GST specific activity was significantly increased to 1.18 fold and 3.34 fold with 2 ppm and 4 ppm of AC, respectively and decreased to 1.77 fold than 3.2 fold of control.
Neurotoxicity of Acrylamide in Developing Chick Embryo
Molecular layer, Purkinje cell neurons and granular layers including white matter between two granular layers were noticed in control cerebellum section as shown in Figure 4. That the both 24 hours and 48 hours intervals, 6 ppm of AC administered brain sections shows granular layer with degenerative changes (GLDC), vacuolation, cerebral hemorrhage and cerebellar layers degeneration and vacuolation and vacuolation between granular layer and molecular layer (V ML & GL) and Purkinje cell necrosis, as shown in Figure 5 and Figure 6, respectively.
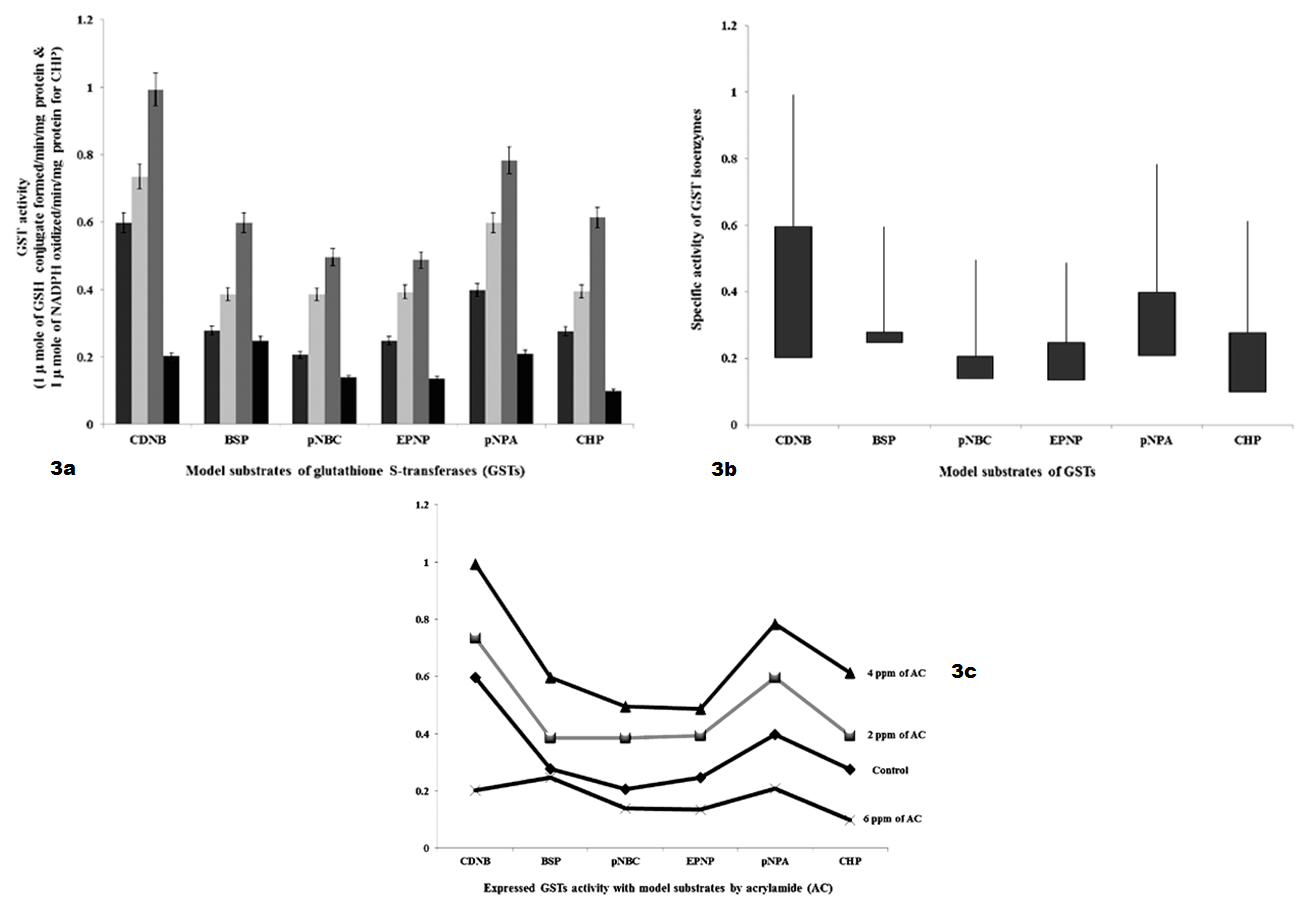
Figure 3 a): GSTs specific activity with model substrates by 48 hours intervening period; b): Differences in GSTs specific activity with model substrates by 48 hours intervening period; c): Expression levels of GST when administered with AC by 48 hours intervening period.
Discussion
As shown in Figure 1, GST expressed to detoxify AC, continuous infiltration and accumulation leads to tissue degeneration. As represented in Table 1, in AC administered chick embryo brain samples, expressed GST isoenzymes were screened by using model substrates. In this study, chick embryo brain GST isoenzymes expressed by the administration of acrylamide (AC) were screened with model substrates like CDNB, BSP, pNBC, EPNP, pNPA and CHP as shown in Table 2 and 3 and Figure 2 and Figure 3 and changes in brain tissue by AC as shown in Figure 5, 6 and control Figure 4.

Figure 4: Chick embryo control cerebellum section with molecular layer, Purkinje cell neurons, granular layers and white matter (H & E stain) (10X).
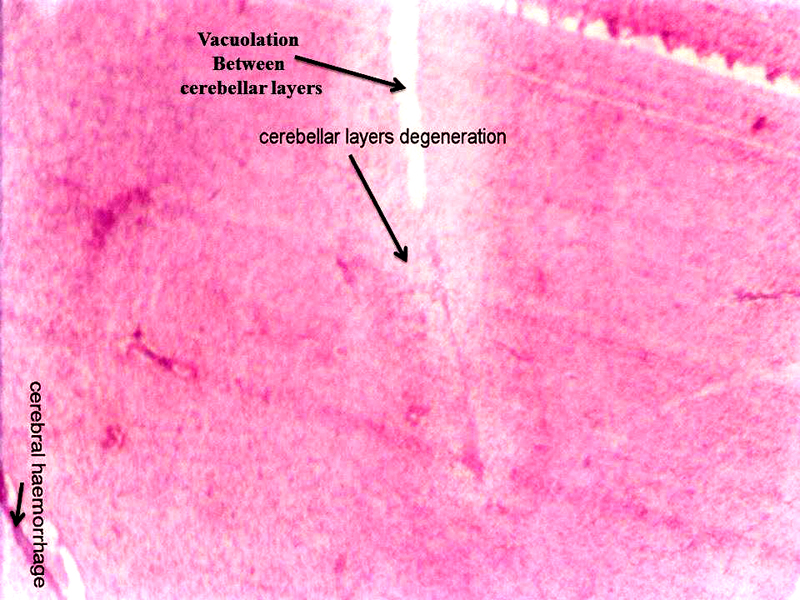
Figure 5: Chick embryo administered with 6 ppm of AC by 24 hours interval, cerebellum section shows vacuolation, cerebral hemorrhage, cerebral layers degeneration (H & E stain) (10X).

Figure 6: Chick embryo administered with 6 ppm of AC by 48 hours interval, cerebellum section shows vacuolation and vacuolation between granular layer and molecular layer (V ML & GL) and Purkinje cell necrosis (H & E stain) (10X).
Mu (µ) class GSTs catalyse the conjugation of GSH with BSP in addition to CDNB (Coombes and Stakelum, 1961; Habig et al., 1974; Mannervik and Jensson, 1982). In review, Hayes and Pulford (1995) said that the mu (μ) GST has shown significant activity with pNBC and EPNP. As shown in Table 2 and 3 and Figure 2 and Figure 3, with the substrate BSP, GST specific activity was significantly increased to 1.6 fold and 1.8 fold by 24 hours interval as well as 1.08 fold and 3.22 fold by 48 hours interval with 2 ppm and 4 ppm of AC, respectively and decreased to 2.2 fold by 24 hours interval and 0.3 fold by 48 hours interval with 6 ppm of AC than 3.2 fold and 2.78 fold of controls, respectively in AC administered chick embryo brain sample. With the substrate CDNB, GSTs activities were significantly increased to 1.8 fold and 2.4 by 24 hours interval as well as 1.34 fold and 4.0 fold by 48 hours interval with 2 ppm and 4 ppm of AC, respectively and decreased to 3.2 fold by 24 hours interval and 4.0 fold by 48 hours interval with 6 ppm of AC than 5.2 fold and 6.0 fold of controls, respectively in AC administered chick embryo brain sample. With the substrate pNBC, GST specific activity was significantly increased to 1.2 fold and 2.3 fold by 24 hours interval as well as 1.85 fold and 5.97 fold by 48 hours interval with 2 ppm and 4 ppm of AC, respectively and decreased to 1.2 fold by 24 hours interval and 0.6 fold by 48 hours interval with 6 ppm of AC than 2.2 fold and 2.0 fold of controls, respectively and with the substrate EPNP, GST specific activity was significantly increased to 1.3 fold and 2.4 fold by 24 hours interval as well as 1.45 fold and 2.39 fold by 48 hours interval with 2 ppm and 4 ppm of AC, respectively and decreased to 0.8 fold by 24 hours interval and 1.12 fold by 48 hours interval with 6 ppm of AC than 2.2 fold and 2.48 of controls, respectively in AC administered chick embryo brain sample. AC treated chick embryo brain GSTs specific activity with BSP, pNBC and EPNP including CDNB shows the existence mu (μ) GST. The present study was agreed with Coombes and Stakelum (1961); Habig et al (1974); Mannervik and Jensson (1982), Hayes and Pulford (1995).
That the alpha (α) GST catalyses the reduction of GSH with CHP (Jakoby and Habig, 1980). It is very interest that the physiological role of non-selenium GPx activity is associated with the GSTs (Burgess et al., 1990). Among GSTs enzymes that the alpha GST can actively protect cells from lipid hydroperoxides which generated by oxidative stress (Spector et al., 2000). Alpha class GST activity towards cumene hydroperoxide (CHP) represents the selenium independent glutathione peroxidase activity (Mannervik et al., 1985; Wendel, 1980). The alpha GST can perform large part of peroxidase activity and this kind of activity is necessary part of defense mechanism of cell (Yang et al, 2002) . As shown in Table 2 and 3 and Figure 2 and Figure 3, with the substrate CHP, GST specific activity was significantly increased to 1.4 and 2.8 fold by 24 hours interval as well as 1.18 fold and 3.34 fold by 48 hours interval with 2 ppm and 4 ppm of AC, respectively and decreased to 2.0 fold by 24 hours interval and 1.77 fold by 48 hours interval than 3.2 fold and 3.2 fold of controls, respectively in AC administered chick embryo brain sample. The present study was agreed with Jakoby and Habig (1980), Burgess et al (1990); Spector et al (2000); Mannervik (1985); Wendel (1980), Yang et al (2002).
A single substrate can gives activity to another class in addition to its specific class (Mannervik et al., 1988). Wild type alpha GSTs activity increased with pNPA and CHP in addition to CDNB than mutant alpha GST (Zhang et al., 2012). Mu GSTs activity enhanced with pNPA and EPNP than genetic variants (Kurtovic et al., 2007). Both alpha GST and Mu GST are present in brain as well as in other organs (Mclellan et al., 1992; Mitchell et al., 1997). It was reported that, based on purification and model substrates specific reaction studies that the CBI and CBII GSTs of chick embryo brain are related to alpha (α) and mu (μ) class GSTs (Dasari et al., 2016). As shown in Table 2 and 3 and Figure 2 and Figure 3, with the substrate pNPA, GST specific activity was significantly increased to 2.6 fold and 4.6 fold by 24 hours interval as well as 2.0 fold and 3.7 fold by 48 hours interval with 2 ppm and 4 ppm of AC, respectively and decreased to 1.4 fold by 24 hours interval and 2.0 fold by 48 hours interval with 6 ppm of AC than 4.4 fold and 4.0 fold of controls, respectively in AC administered chick embryo brain sample. This present study was agreed with Mannervik et al (1988); Jakoby and Habig, (1980); Zhang et al. (2012); Kurtovic et al (2007); Mclellan et al (1992); Mitchell et al (1997).
Acrylamide shows marked efficiency binding to brain (Sumner et al., 1997). In both laboratory animals and humans, neurotoxicity of AC is characterized by ataxia and distal skeletal muscle weakness (Yu et al., 2006). Brain, spinal cord and sciatic nerve affects to the oxidative stress of AC in nervous system including sensory and motor dysfunction in rats (Zhu et al., 2008). That the brain histopathological study revealed neuronal degeneration, separation of fibers and necrosis were noticed (Jangir et al., 2016). As shown in the Figure 5 and Figure 6, AC administered chick embryo brain tissue was seriously injured when compared with control Figure 4. This shows the similar result of Jangir et al (2016).
Conclusion
Mainly, CBI and CBII GSTs (our published work) were expressed in response to acrylamide (AC) toxicity in developing brain of chick embryo. High level expression of those GSTs and GST associated peroxidase (Gpx) activity can protect the brain from AC toxicity up to some extent. Due to the continuous infiltration of toxic chemical agents like AC finally made injury to brain tissue. In conclusion, CBI and CBII GSTs of chick embryo brain are very similar to alpha (α) class and mu (µ) class GSTs and these protein biomarkers can protect the developing brain from environmental toxic chemical agents.
Materials and Methods
Chemicals
Glutathione (reduced) (GSH), acrylamide (AC) 99.9%, 1-chloro 2, 4-dinitro benzene (CDNB), Bromosulfopthalein (BSP), p Nitrobenzyl chloride (pNBC), 1, 2-Epoxy 3(p-nitro penoxy propane (EPNP), p-Nitrophenyl acetate (pNPA) were purchased were purchased from Sigma Chemical Company, St. Louis, MO, USA.
Ethylenediaminetetraacetic acid (EDTA), glacial acetic acid, Cumene hydroperoxide (CHP), Hydrochloric acid (HCL), hydroxymethyl aminomethane (Tris base), glycine, glycerol, formaldehyde, phenylmethanesulphonyl fluoride (PMSF), sodium azide, Sodium Potassium tartrate, Sodium Chloride (NaCl), Potassium chloride (KCl) were purchased from SD fine chemicals, Mumbai, India and all other chemicals were procured from the local companies with high quality.
Maintenance of Eggs and Route of Chemical Administration
Fertilized Babcock strain chicken eggs weighing about 50 grams were incubated at 370 C, maintain 60% humidity and rotated them for every three hours. Eggs were treated with acrylamide on day 12th and 15th of incubation, under sterile conditions. Control eggs were injected with vehicle (distilled water) only. A hole was made in the shell at the blunt end of the egg and a micro syringe was inserted through the air sac, onto the inner shell membrane, where 2 ppm to 10 ppm AC was deposited. The inner shell membrane has direct contact with the chorio-allantoic membrane. This route of administration gives faster uptake of the substances than yolk injections. During this process, the survival embryos were tested using candler light. The dead eggs were discarded and survived eggs were selected for further experiment ation.
Tissue Collection and Sample Preparation
Brain tissue was collected from both normal and treated embryo on 18th day by pressing small head with forceps and washed with cold 50 mM Tris HCl buffer (pH 8.0), containing 1mM ethylenediaminetetraacetic acid (EDTA) in order to remove excess blood and body fluids. Instantly collected tissue was preserved at -20ºC for further experimentation. At the time of experimentation, the brain tissue was slightly thawed and 20 % homogenate was prepared in 50 mM Tris-HCl buffer (pH 8.0), containing 0.25M sucrose and 1mM phenylmethanesulphonyl fluoride (PMSF) using a potter Elvijhem homogenizer. Homogenization was done by keeping the potter Elvijhem homogenizer in an ice jacket and care was taken to minimize the froth formation. The homogenate was passed through two layers of cheese cloth to remove floating lipid materials and the resulting supernatant was centrifuged at 10,000 rpm by two times for 45 minutes at 4ºC. The collected supernatant was known as cytosolic fraction and it was used as the enzyme source for activity assays.
Assay of Glutathione S-Transferase (Gst) Activity
Conjugation of reduced GSH to 1-chloro-2,4-dinitrobenzene (CDNB) was stimulated by the addition of enzyme source as described by Habig et al (1974). Briefly, 100 μl enzyme source was added to cuvettes containing 1ml phosphate buffer (pH 6.5), 100 μl 30 mM CDNB and 100 μl 30 mM GSH and final volume adjusted to 3 ml. Change in absorbance at 340 nm was measured over a span of 3 min to calculate the rate of conjugation of CDNB and expressed as µ mole CDNB-GSH conjugate formed /min/mg protein.
Specific Activity Assay of Glutathione S-Transferases (Gsts)
GST isoenzymes in both control and AC treated chick embryo brain cytosolic fraction were screened by classical substrates such as Bromosulfophthalein (BSP), p-Nitrophenyl acetate (PNPA) and Cumene hydroperoxide (CHP) in addition to 1-chloro 2, 4-dinitro benzene (CDNB by the method of Habig and Jakoby (1981) and Wendel, (1981) respectively.
Estimation of Protein
Protein concentration was determined in both control and treated cytosolic fractions including affinity purified GST sample by the method of Lowry et al (1951).
Histopathological Studies
According to Humason (1979), brain tissue histological examinations were conducted. Briefly, collected brain tissues from both control and experimental embryos were washed with physiological saline (0.9% NaCl) to remove blood and fat debris adheres to the brain. After fixation in 10% of formalin the tissues were allowed to process. In first step the tissues were washed under running tap water to remove the fixative. In second step, tissues were allowed for dehydration by a graded series of alcohol and the tissues were allowed to clear by using methyl benzoate and subjected to embed in paraffin wax. In third step, the tissue were subjected to cutting with 6 µ thickness and such sections allowed for staining with haematoxylin and eosin (H & E). In fourth step, the sections were mounted with Canada balsam and observed under light microscope.
Statistical analysis
All the data related to this study and their results were calculated from three experiments and presented as the mean ± standard deviation (SD). Student t-test was performed to identify the acrylamide treated brain samples differed from the mean for the respective vehicle controls. That the differences between the experimental groups at the level of P <0.05 were considered as significant.
Acknowledgments
We are grateful to the University Grants Commission (UGC), New Delhi, India for generous financial assistances including JRF and SRF (ROC. No.20601/UGC-1/2/RGNF/2007).
Conflict of interest
Authors do not have any potential conflict of interest.
AUTHORS CONTRIBUTION
All authors equally contributed to this article.
References