Advances in Animal and Veterinary Sciences
Research Article
Characterization of Ulva fasciata Ethanolic Extract-mediated Biosynthesized Silver Nanoparticles and Evaluation of their Nephrocadiopreventive Effects in Doxorubicin-injected Wistar Rats
Asmaa M. Mahmoud1, Basant M. Mohamed1, Ibraheem B.M. Ibraheem2, Hanan A. Soliman1, Osama M. Ahmed3*
1Biochemistry Department, Faculty of Science, Beni-Suef University, P.O. 62521, Beni-Suef, Egypt; 2Botany and Microbilogy Department, Faculty of Science, Beni-Suef University, P.O. 62521, Beni-Suef, Egypt; 3Physiology Division, Zoology Department, Faculty of Science, Beni-Suef University, P.O. 62521, Beni-Suef, Egypt.
Abstract | The aim of this study was to assess the preventive effects of Ulva fasciata (U. fasciata) ethanolic extract and silver nanoparticles (AgNPs) biosynthesized by this extract against nephrocardiototoxicity caused by doxorubicin in male Wistar rats. Biosynthesized AgNPs were also characterized and the constituents of U. fasciata ethanolic extract were identified. To assess the renocardiopreventive effects, male Wistar rats were orally treated with of U. fasciata ethanolic extract and its biosynthesized AgNPs (100 mg/kg body weight; 3 times/week) concurrently with intraperitoneal injection of doxorubicin (2.5 mg /kg body weight three days/week) for 6 weeks. Renocardiopreventive effects of U. fasciata extract and AgNPs/U. fasciata were manifested by decrease in the elevated serum levels of kidney biomarkers (creatinine, urea and uric acid) and the elevated serum activities of cardiac enzymes (CK-MB, AST and LDH) together with the improvement of kidney and heart bioindicators of oxidative stress and antioxidant defense system as well as kidney and heart histological changes; the AgNPs/U. fasciata was more effective. In conclusion, U. fasciata extract and AgNPs/U. fasciata, which is more potent, could have preventive effect against doxorubicin-induced renocardiotoxicity in Wistar rats and this effect could be mediated, at least in part, via enhancement of the antioxidant defense system.
Keywords | Doxorubicin, Ulva fasciata, Silver nanoparticles, Nephrotoxicity and cardiotoxicity
Received | May 31, 2020; Accepted | August 15, 2020; Published | September 20, 2020
*Correspondence | Osama M. Ahmed, Physiology Division, Zoology Department, Faculty of Science, Beni-Suef University, P.O. 62521, Beni-Suef, Egypt; Email: osamamoha@yahoo.com; osama.ahmed@science.bsu.edu.eg
Citation | Mahmoud AM, Mohamed BM, Ibraheem IBM, Soliman HA, Ahmed OM (2020). Characterization of Ulva fasciata ethanolic extract-mediated biosynthesized silver nanoparticles and evaluation of their nephrocadiopreventive effects in doxorubicin-injected wistar rats. Adv. Anim. Vet. Sci. 8(s2): 98-111.
DOI | http://dx.doi.org/10.17582/journal.aavs/2020/8.s2.98.111
ISSN (Online) | 2307-8316; ISSN (Print) | 2309-3331
Copyright © 2020 Mahmoud et al. This is an open access article distributed under the Creative Commons Attribution License, which permits unrestricted use, distribution, and reproduction in any medium, provided the original work is properly cited.
Introduction
Doxorubicin (Adriamycin) is an anthracycline anticancer drug that is commonly applied for the chemotherapy of solid and hematological tumors such as breast cancer, soft-tissue sarcomas, osteosarcomas, leukemia and lymphomas (Cappetta et al., 2017). However, its clinical use may be restricted due to its severe toxicological history in various organs including kidneys and heart (El-Sherbiny and ElSherbiny, 2014; Pugazhendhi et al., 2018; Mohajeri and Sahebkar, 2018; Zhao et al., 2018a). Several studies have indicated that doxorubicin-mediated toxicity is a result of oxidative stress, DNA damage, lipid peroxidation, apoptosis and induced autophagy and mitochondrial injury (Zhao et al., 2018b). Many other publications reported nephrotoxicity and cardiotoxicity effects of doxorubicin in experimental animals (Ahmed et al., 2017; Kaiserová et al., 2017; Nagai et al., 2018; Ahmed et al., 2019, 2020). These publications stated the crucial implication of oxidative stress and antioxidant defense system in inducing the nephrocardiotoxicities. Based on these findings, the use of natural antioxidant or green biosynthesized nanoparticles that enhance the antioxidant efficiency of bioactive ingredients may suppress or abrogate the side effects and toxicity of the chemotherapeutic drugs like doxorubicin.
Silver nanoparticles (AgNPs) are of great significance to biomolecular catalysis, electron transfer and oxygen transportation as a result of the reaction with amino acids and proteins as the main targets of medicines in the organisms (Wang et al., 2007; Cai et al., 2010). Nowadays, AgNPs has efficient biological action due to its physicochemical property in which larger surface to volume ratio resulted into higher surface exposure (Nadhe et al., 2019). Green synthesis of AgNPs using plants and algae are ecofriendly procedures without need to the use of high-cost and toxic chemicals (Javaid et al., 2018; Nadhe et al., 2019). Marine algae have valuable contents such as polysaccharides (alginates, laminarians and fucans) and other pharmaceutical and nutraceutical compounds (Rahimi et al., 2014). They were used to mediate reduction of silver into AgNPs (Anand and Mandal, 2015). The green macroalga, U. fasciata, had potent therapeutic value and it was used for synthesis of AgNPs (Rao and Boominathan, 2015). AgNPs synthesis by U. fasciata had toxic effects against cancer (El Bialy et al., 2017; Hamouda et al., 2019) and very high antibacterial activity (Rao and Boominathan, 2015).
U. fasciata Delile, which belongs to phylum Chlorophycota, class Ulvophyceae, order Ulvales and family Ulvaceae (Valeem and Shameel, 2006), is a dark green or bright grass green in colour and may be colorless under stressed conditions. The Thallus is thin and sheet like, consist of broad blades, basal portion 10-15 cm wide, tapering at the base, up to 15 cm lengthy, broad at the base and joint, but upper part is divided deeply into many ribbon like segments, margin soft, often undulate and holdfast is small. U. fasicata has shown antioxidant (Kokilam and Vasuki, 2014), antileishmanial (Sabina et al., 2005), hypoglycemic (Subash-Babu et al., 2008; Abirami and Kowsalya, 2015), antibacterial (Selvin et al., 2004; Shahnaz and Shameel, 2006), antifungal (Langeswaran et al., 2019), nematicidal and insecticidal activities (Valeem et al., 2011).
Therefore, the present study used U. fasciata ethanolic extract for biogenic synthesis of AgNPs and aimed to assess the antagonistic effects of the U. fasciata ethanolic extract and its biogenic AgNPs on doxorubicin-induced renocardiotoxicities in Wistar rats.
MATERIALS AND METHODS
Alga collection and isolation
U. fasciata was collected on March 2014 from the shallow water beside of the Mediterranean Sea shores at Abo Qir coast, Alexanderia, Egypt. The identification and taxonomy of the seaweed samples was carried out by Prof. Dr. / Borie M. Ibraheem (Professor of Microbiology, Botany and Microbiology Department, faculty of science, Beni-Suef university, Egypt).
Preparation of algal extract
The collected alga was washed with marine water, then with tap water and finally with distilled water. The washed alga was blotted, spread out and dried in good aerated shade area at room temperature for two weeks. Shade dried sample was ground to fine powder by an electric grinder. The powdered sample was stored in sterilized containers for further usage. Powdered sample of U. fasciata (100 g) were extracted with 400 ml of absolute ethyl alcohol for 6–8 hours (hrs) in Soxhlet apparatus (500 mL boiler). At the end of extraction, the alga debris in extract was filtered out and the ethanol in the extract was evaporated to viscous semi-solid residues in a vacuum at 35 ᵒC using a rotatory evaporator. At the end, the dried extract was stored at 4 ᵒC for pending its use for analysis and treatment.
Synthesis of AgNPs
It was done by adding 10 ml of U. fasciata ethanolic extract to 90 ml of 1 mM of AgNO3 at room temperature. At once, the color changed to greenish yellow color on mixing of AgNO3 solution to algal extract. Then, the color changed bit by bit until it became dark brown color which indicated the formation of AgNPs (Ibraheem et al., 2016).
Characterization of synthesized AgNPs
Visual characterization
Biosynthesis of AgNPs was verified by observation of the developed color in the reaction as a result of the algal extract with 1 mM AgNO3 solution in comparison with the control. The color changed from pale yellow to brown indicating the biogenesis of AgNPs. The biogenic AgNPs were obtained by centrifugation at 12000 r.p.m. for 10 min. The pellets were washed twice with distilled water. This protocol was repeated thrice. Afterthat, the AgNPs pellets were lyophilized for further uses.
Ultra Violet–Visible (UV-Vis) spectroscopy analysis
The UV-spectroscopy of biogenic AgNPs was examined according the methods of Swamy et al. (2015), Abdel-Raouf et al. (2017) and Hamed et al. (2017).
Transmission Electron Microscopy (TEM) analysis of AgNPs
The morphological analysis of the NPs was achieved by TEM according to the methods of Abdel-Raouf et al. (2017), Hamed et al. (2017) and Hamouda et al. (2018).
Characterization analysis of U. fasciata extract
Fourier transform infrared analysis (FTIR)
FTIR was used to identify the possible biomolecules responsible for the reduction of the Ag ions and capping of the bioreduced AgNPs synthesized by U. fasciata extract according to the method of Hamed et al. (2017).
Gas Chromatographic/Mass Spectrometric (GC-MS) Analysis
GC-MS analysis was performed according the methods of Rodeiro et al., (2015).
Chemicals
Doxorubicin (Adriblastina) was obtained from Carlo Erba, Turkey in the form of 10 mg/ampoule. Silver nitrate (AgNO3) and absolute ethyl alcohol was obtained from Sigma Chemicals Company, St. Louis, MO, USA. All other chemicals used for the investigation were of analytical grade and were commercially obtained.
Experimental animals
Male rats of Wistar strain, weighing between 100-120 g and aging 9-11 weeks, were used in the present investigation. They were obtained from the Animal House of Abou Rawash, El-Giza, Egypt. They were maintained under strict care and observation for 2 weeks before the starting the experiment to exclude any intercurrent infection. The chosen animals were housed in polypropylene cages with good aerated stainless steel covers at temperature of 25±5ºC and normal 12-hours daily light-dark cycle. The rats were supplied with access of water and standard balanced diet ad libitum. All animal procedures were approved by the Experimental Animal Ethics Committee, Faculty of Science, Beni-Suef University, Egypt (Ethics approval number: BSU/FS/2017/18).
Doses and treatment
The animals were injected with doxorubicin by an intraperitoneal route (i.p.r.) in a dose level of 2.5 mg/kg b. wt three days/week for 6 weeks. The doxorubicin was dissolved in 0.9 % NaCl (Kabel et al., 2018; Pugazhendhi et al., 2018). The dose of U. fasciata and AgNPs of U. fasciata used in this study was 100 mg/kg b. wt (Margret et al., 2009) which was given 3 times per week by oral gavage for 6 weeks. They were dissolved in 1% CMC as a vehicle at concentration 100 mg U. fasciata and AgNPs/U. fasciata/5 ml 1% CMC.
Experimental design
Forty rats were used in the present study. These rats were divided into 4 groups (n = 10) designed as follow:
Group 1 (Normal control): The rats within this group were supplemented with the equivalent volume of vehicles 0.9% NaCl by i.p.r. and 1% CMC 3 times/week by oral gavage for 6 weeks.
Group 2 (Doxorubicin–injected control): The rats within this group were injected with doxorubicin in a dose level of 2.5 mg/kg b. wt by i.p.r. 3 times/week for 6 weeks.
Group 3 (Doxorubicin-injected rats treated with U. fasciata extract: The rats within this group were injected with doxorubicin in a dose level of 2.5 mg/kg b. wt by i.p.r. 3 times/week for 6 weeks and were orally treated with algal extract in dose level of 100 mg/kg b. wt 3 times/week by oral gavage for 6 weeks.
Group 4 (Doxorubicin-injected rats treated with U. fasciata AgNPs: The rats within this group were injected with doxorubicin in a dose level of 2.5 mg/kg b. wt. by i.p.r. 3 times/week for 6 weeks and were orally treated with U. fasciata AgNPs at dose level of 100 mg/kg b. wt. 3 times/ week by oral gavage for 6 weeks.
Sampling and tissue preparation
By the end of the experiment, six animals of normal, doxorubicin-injected control rats and doxorubicin-injected rats treated with U. fasciata and U. fasciata biosynthesized AgNPs were sacrificed under mild diethyl ether inhalation anesthesia. Blood samples were collected from jugular vein in serum-separating gel and clot activator tubes. Tubes containing blood were centrifuged at 3000 rpm. at 30 ºC for 15 minutes and sera were aspirated into Eppendorf tubes. The sera were frozen at -30ºC pending biochemical analyses. Kidney and heart from each animal were rapidly dissected out and were perfused in sterile saline. One kidney and one half of heart were fixed in neutral buffered formalin for 24 hours and then transferred into 70% alcohol for histopathological examination. The other kidney and the half of heart were separately homogenized into 0.9% sterilized NaCl (10% w/v) by Teflon Homogenizer (Glas-Col, Terre Haute, USA).
Biochemical analyses
Creatinine levels in sera were assayed based on the procedure of Henry (2001) using the reagent kits obtained from Diamond Diagnostics, Egypt. Urea levels in sera were detected based on the procedure of Vassault et al. (1986) using the reagent kits purchased from Biomed Diagnostics, Egypt. Uric acid levels in sera were estimated based on the method of Tiffany et al. (1972) by reagent kits purchased from spectrum Company, Egypt. Creartine kinase-MB (CK-MB) activity was assayed by kinetic method using kits developed by Spectrum Diagnostics, Egypt based on the method of Friedman and Young (1997). Aspartate aminotransferase (AST) activity in serum was detected by kinetic method according to the method of Burtis et al. (2005) using reagent kits obtained from BioSystem Company (Spain). Lactate dehydrogenase (LDH) activity in serum was estimated by kinetic method according to the method of Witt and Trendelenburg (1982) using reagent kits purchased from Human (Germany).
Kidney and heart GSH (glutathione) contents were quantified based on the method of Beutler et al. (1963). Liver lipid peroxidation was quantified in kidney and heart by measuring manlondialdehye (MDA) production based on the procedure of Preuss et al. (1998). GST (glutathione-S-transferase) activities in kidney and heart were detected base on the publication of Mannervik and Gutenberg (1981). GPx (glutathione peroxidase) activities in kidney and heart were determined based on Tappel (1978).
Histopathological studies
Fixed kidney and heart tissues samples were transported to Pathology department, NCI (National Cancer Institute), Cairo University, Egypt for processing and staining of 5µm thick sections with hematoxylin and eosin (H and E) for histopathological examination.
Statistical analysis
Data are represented as mean ± standard error mean (SEM). Analysis of variance (one way-ANOVA) was used to identify statistically significant differences between groups. One way-ANOVA was followed by least significant difference (LSD) to compare various groups at P<0.05. All statistical analyses were performed using SPSS 15.0 software.
RESULTS AND DISCUSSION
Characterization of synthesized AgNPs and identification of chemical constituents of the extract
Visual characterization
Before adding alga ethanolic extract to AgNO3 solution, algae extract had green color and AgNO3 solution was colorless. After addition of the alga extract to AgNO3 solution, the color gradually changed to dark brown (Figure 1). At once after addition, the color changed to yellow and by the time, it changed to the dark brown color which indicated the formation of AgNPs (Figure 2).
Reduction of AgNO3 was visually evidenced by the color change (colorless to brownish yellow) of reaction mixture after 3 minutes of reaction (Figure 2). The increase in the intensity of brown color is in direct proportional to the period of incubation. The change in color to brown may be attributed to the excitation of surface plasmon resonance (SPR) effect and reduction of AgNO3 (Mulvaney, 1996). Also, the time taken for AgNPs formation is very fast (less than 3 minutes). These results agree with Rajeshkumar et al. (2012) and Abdel-Raouf et al. (2013) who reported that the appearance of dark brown color in solution. Also, Ajitha et al. (2015) reported the formation of AgNPs in a few minutes. Otherwise, Bhimba et al. (2014) and Devi and Bhimba (2014) suggested the time formation of AgNPs 10 minutes at 121ºC. In disagreement with the present study, Rahimi et al. (2014) reported that the formation of AgNPs took long time Furthermore, Parveen and Lakshmi (2016) reported that in the biosynthesis of AgNPs using red alga, Amphiroa fragilissima, the time of reduction of AgNO3 was 20 minutes while in the current work, the algal NPs recorded high stability and formed within short time.

Figure 1: Formation of the dark brown AgNPs after adding algal ethanolic extract. (a) represents the clear AgNO3 (0.001M) solution. (B) represents the algal ethanolic extract. (C) Biosynthesized AgNPs.
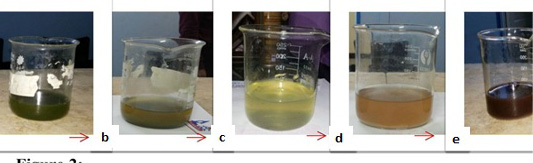
Figure 2: Dark brown color of the biosynthesized AgNPs with time. (a) represents the clear U. fasciata ethanolic extract. (b) represents greenish yellow color at once after adding 10 ml of algal extract to 90 ml 0.001 M of AgNO3 at room temperature. c: represents yellowish color. (d) represents brownish color. (e) represents the solutions that change gradually till become dark brown color which indicates the formation of AgNPs with the time.
UV-visible spectroscopy analysis
The formation of AgNPs was confirmed by changes in color followed by UV-Visible spectrophotometric analysis which has been shown to be a very useful technique for the analysis of some metal NPs.
The biogenesis of AgNPs was confirmed by a UV-Visible spectral scan at a wavelength ranged from 200 to 800 nm. SPR spectrum of AgNPs produced by using U. fasciata ethanolic extract depicted a distinct absorption peak at 210 nm and the other at 659.50 nm (Figure 3) that prove the presence of AgNPs. Brause et al. (2002) demonstrated that optical absorption spectra of metallic NPs is predominantly dominated by SPR and the peak of absorption correlated with particle size. SPR peak of AgNPs in aqueous dispersion shifts to longer wavelengths with increase in particle size. The position and shape of plasmon absorption of AgNPs depend on many indices including particle size, shape and the dielectric constant of the reaction medium as well as particles adsorbed on surfaces of AgNPs (Krishnaraj et al., 2010).
FT-IR analysis
FT-IR spectroscopy measurements were performed to recognize the possible biomolecules found in U. fasciata ethanolic extract which are responsible for reduction and capping of AgNPs.
FT-IR spectrum (Figure 4) shows the molecular configuration of different functional groups present in ethanolic extract of U. fasciata showed bands at 3391.661 (cm-1) assigned to N-H stretche, at 2932.978 (cm-1) assigned to C-H Stretch, at 2385.984 (cm-1) assigned to P-H, at 2270.329 (cm-1) assigned to N=C=O, at 1644.523 (cm-1) assigned to C=C group, at 1429.147(cm-1) assigned to C=C group, at 1103.149(cm-1) assigned to C-O group and at 626.527(cm-1) assigned to C-Cl group. These stretched bands refer to presence of some functional groups such as 1ry and 2nd amine and amide, alkane, phosphine, isocyanate, alkene, aromatic, alcoholic and alkyl halide, respectively. Similar findings pertaining to other marine algea were reported earlier by several other researchers (Manilal et al., 2010; Anbarasan et al., 2013; Trivedi et al., 2013; Ibraheem et al., 2016; Zaid et al., 2016; Khatri et al., 2017).
The main band at 3391.661 (cm-1) assigned to 1ry, 2nd amine and amide N-H stretched band and this indication for protein prescence. Proteins most possibly could form a coat cover of the metal NPs (i.e., capping of AgNPs) to prevent agglomeration of the particles and stabilizing in the medium (Sahayaraj and Rajesh, 2011). Also, proteins represent another important utility of this feedstock as a potential source of proteinaceous diet (Wassef et al., 2013). Also, U. fasciata contains other bands such C-H, C=C and C-O. These groups showed for presence of polysaccharide, carbohydrates of monosaccharide and biological molecules such as secondary metabolites found on marine algae (Sahayaraj and Rajesh, 2011; Shiny et al., 2013). These groups could possibly play important role in the synthesis and stabilization of metal NPs.
TEM analysis of AgNPs
TEM of AgNPs analysis, measurement was applied to characterize the NPs size and morphology. TEM micrographs of representative AgNPs synthesized by U. fasciata ethanolic extract are shown in Figure 5. These results showed that U. fasciata ethanolic extract strongly affected the size and shape of the AgNPs weren’t uniform in size and shape. The NPs were large and small spherical particles and small percentages of rod. TEM analysis of particles size also showed maximum particles in size 2 to 200 nm.

Figure 5: Representative TEM micrograph AgNPs synthesized by the reduction of AgNO3 ions in U. fasciata ethanolic extract.
TEM has provided further insight into the size details and the morphology of the biosynthesized AgNPs. TEM images and histograms of particle size distribution recorded from AgNPs solutions are shown in Figure 5. It is observed that the biogenic NPs are polydispersed and predominantly spherical in shape (Dhas et al., 2014) with maximum diameter size of 200 nm. All the nanoparticles are well separated and no agglomeration was observed (El-Rafie et al., 2013). Bioorganic materials bound to the nanoparticles surfaces which are responsible for the stability of nanoparticles (Amaladhas et al., 2012).
Read time (RT min) | Chemical compound | Nature of compound | Peak area % | Biological activity |
3.285 | Cabamic acid, methyl ester (urethylane) | Ester | 1.61% | Antiparasitic activity and antifungal |
3.675 | Furfural (2-furanecarboxyldhyde) | aldehyde | 2.20% | Mutagenic and anticarcinogenic |
4.374 | Sulfonylbismethane (DMSO) (Dimethulsulfoxide) | organosulfur compound | 20.87% |
anti-inflammatory antioxidant and |
7.192 | 1-Ethyl-4-methylbenzene (toluene) | Aromatic | 0.7% | Antioxidant |
10.185 | Methyl-2-butenylether | Flavonoids | 0.58% | Antimalarial |
11.780 | Dodecane (Bihexyl) | alkane hydrocarbon | 0.34% | Antimicrobial |
15.244 | Cyclohexasiloxane (Dodecamethyl-CAS) | Cosmetic | 0.37% | Antimicrobial |
17.055 | Tetradecane (Isotetradecane) | Iodo (alkane) | 0.81% | Antimicrobial |
20.455 | (7aR)-5,6,7,7a-Tetrahydro-4,4,7atrimethyl (2(4H)-benzofuranone) | Terpenes | 1.43% | antimicrobial activities |
21.852 | Hexadecane (n-cetane) | Hydrocarbon | 1.29% | Antiviral and antimicrobial |
23.628 | 8-heptadecene (Heptadec-8-ene) | Alkene | 3.31% | antimicrobial and antibacterial |
24.688 | Tetradecanoic acid (Myristic acid) | Fatty acid | 0.18% | Antimicrobial and antimalarial |
25.972 | Loliolide | Monoterpene lactone | 1.00% | antioxidant, ANT-REPELLENT, antimicrobial |
26.184 | Octadecane (n-octadecane) | Alkane | 0.58% | antimicrobial and antioxidant antibacterial |
28.356 | Hexadecanoic acid methyl ester (methyl palmitate) | Fatty acid methyl ester | 11.03% | Anti-oxidant, decrease blood cholesterol, anti-inflammatory |
39.447 |
1,2 Benzene dicarboxylic acid |
Ester | 0.38% | Antimicrobial, antifungal, anti-malarial |
24.688 |
Tetradecanoic acid methyl |
Fatty acid methyl ester | 0.32% | Antibacterial, antifungal |
29.841 |
Hexadecanoic acid |
Fatty acid | 11.77% | Antimicrobial Antioxidant anti-inflammatory and anti-mutagenic. |
30.085 | Hexadecanoic acid, ethyl ester (ethyl palmitate) | Ester of fatty acid | 1.34% | Antioxidant, hypocholesterolemic nematicide, pesticide, anti androgenic flavor, hemolytic, 5-Alpha reductase inhibitor |
32.056 | 9,12,15-Octadecatrienoic acid, (Z,Z,Z) methyl ester | Fatty acid of methyl ester | 1.84% | Antiinflammatory, hypocholesterolemic cancer preventive, hepatoprotective, nematicide, insectifuge, antihistaminic antieczemic, antiacne, 5-Alpha reductase inhibitor, antiandrogenic, antiarthritic, anticoronary, insectifuge |
27.017 | Neophytadiene (2-hexadecene-1-ol) | Terpenes | 4.51% | Antimicrobial |
32.158 | 9-Octadecenoic acid (Z) methyl ester (methyl elaidate) |
Unsaturated fatty acid |
1.31% | Antioxidant, anti cancer |
27.518 | 2-Hexadecen-1- ol (3,7,11,15-tetramethyl-RRR- (E)-(T)- Phytol) | Terpene alcohol | 4.22% | Antimicrobial anti-inflammatory anticancer diuretic |
26.184 | Octadecane (n-octadecane) | Alkane | 0.58% | Antimicrobial |
33.334 | Oleic acid (9-octadeconic acid) | Fatty acid | 2.62% | Antimicrobial |
32.513 | Octadecanoic acid methyl ester (methyl stearate) | Fatty acid methyl ester | 0.73% | Antimicrobial |
27.162 | 2-Pentadecanone (hexahydrofarnesyl acetone) | Ketone | 0.67% |
Antimicrobial
|
GC-Ms analysis of the ethanolic extract of U. fasciata
Marine macroalgae are one of nature’s most biologically active resources, as they possess multiple bioactive compounds. Compounds isolated from marine macroalgae have exerted many biological activities (Babu et al., 2014).
The GC-MS analysis of U. fasciata showed presence of 27 bioactive compounds; these compounds are presented in Table 1. The main compounds in this sample were DMSO with peak area (20.87%), palmitic acid with peak area (11.77%), methyl palmitate with peak area (11.03%), Neophytadiene with peak area (4.51%) and 2-Hexadecen-1- ol (3,7,11,15-tetramethyl-RRR- (E)-(T)- Phytol with peak area (4.22%). The confirmation of the presence of compounds increase the knowledge on the phytochemical composition of U. fasciata ethanolic extract and it must add to the understanding of the product pharmacological properties (Rodeiro et al., 2015). Phytochemical components including flavonoids, tannins, aromatic compounds or secondary metabolites act as defense mechanism against many microorganisms. The therapeutic properties of green algae and medicinal plants may be due to the presence of several secondary metabolites such as flavonoids, alkaloids, saponins, tannins, phenolic compounds and phytosterols (Britto et al., 2012; Khatri et al., 2017).
Effects on biochemical and oxidative stress parameters
The kidney is one of the main target organs subjected to toxic xenobiotics because they receive large blood flow (about 21% of cardiac output) and has a capacity to extract and concentrate toxic materials by its highly specialized cells (Azab et al., 2014). Data in Table 2 indicated that serum creatinine, urea and uric acid levels significantly (p<0.05) elevated in doxorubicin-injected rats in comparison to control group; the recorded percentage increases were 16.67%, 159.38% and 56.25%, respectively. These data are in accordance with those obtained by many authors (Yilmaz et al., 2006; Alberto et al., 2008). The increase in these parameters in serum reflects the impairment of kidney function (Varely, 1987; Bedi and Priyanka, 2012; Ahmed et al., 2017, 2019) as a result of doxorubicin intraperitoneal injection in a dose level of 2.5 mg/kg b. wt 3 times/week for 6 weeks. On the other hand, the doxorubicin-injected rats treated with U. fasciata and AgNPs/U. fasciata showed a significant decrease (p<0.05) in serum creatinine and urea levels. Thus, these results reflect the effective nephropreventive effects of both treatments in doxorubicin-injected rats. While AgNPs/U. fasciata produced a significant decrease in serum uric acid level, U. fasciata induced a non-significant effect (p>0.05) in comparison with doxorubicin-injected control. AgNPs/U. fasciata was more potent than U. fasciata extract in decreasing serum creatinine and uric acid levels. These results are agreement with Mahmoud and Hussein (2014) who demonstrated that aqueous extract of the green macroalga, Ulva lactuca, reduced the nephrotoxicity against N-nitrosodiethylamine and phenobarbital and AbdEl-Raouf et al. (2017) who reported the anti-nephrotoxicity of biogenic AgNPs synthesized by U. fasciata. In addition, BelHadj et al. (2013) had also shown that Ulva lactuca polysaccharides produced a decrease in plasma creatinine and urea levels; thus preventing kidney dysfunctions.
Table 3 indicated that serum CK-MB, AST and LDH activities exhibited a significant (P<0.05) increase in doxorubicin-injected rats in comparison to control group; the recorded percentage increases were 44.59%, 143.79% and 578.17% respectively as compared with corresponding normal controls. These results are in accordance with Bast et al. (2007), Ahmed et al. (2017), Chen et al. (2017), Nimbal and Koti (2018) and Ahmed et al. (2019).
Table 2: Effect of U. fasciata ethanolic extract and AgNPs/U. fasciata on serum creatinine, urea and uric acid levels in doxorubicin-injected rats.
Parameters | Creatinine (mg/dl) | % Change | Urea (mg/dl) | % Change | Uric acid (mg/dl) | % Change |
Groups | ||||||
Normal |
0.60 ± 0.02b |
- |
12.80 ± 0.36a |
- |
1.60 ± 0.08b |
- |
Doxorubicin |
0.70 ± 0.02c |
16.67 |
33.20 ± 0.95c |
159.38 |
2.50 ± 0.17c |
56.25 |
Doxorubicin + U. fasciata |
0.60 ± 0.02b |
-14.29 |
24.50 ± 1.25b |
-26.20 |
2.40 ± 0.09c |
-4.00 |
Doxorubicin + AgNPs/U. fasciata |
0.50 ± 0.04a |
-28.57 |
25.10 ± 1.03b |
-24.40 |
1.20 ± 0.09a |
-52.00 |
LSD at the 5% level | 0.04 | 1.44 | 0.15 | |||
F- probability | P<0.001 | P<0.001 | P<0.001 |
Data are expressed as Mean ± SE; Numbers of detected samples in each group is six; Means, which have different superscript symbol, are significantly different at p<0.05; Percentage changes were calculated by comparing doxorubicin-administered group with normal control and doxorubicin-administered groups treated with U. fasciata ethanolic extract and AgNPs/U. fasciata with doxorubicin-administered control groups.
Table 3: Effect of U. fasciata ethanolic extract and AgNPs / U. fasciata on serum CK-MB, AST and LDH activitie in doxorubicin-injected rats.
Parameters | CK-MB (U/L) | % Change | AST (U/L) | % Change | LDH (U/L) | % Change |
Groups | ||||||
Normal |
370.00 ± 13.10a |
- |
44.30±2.00a |
- |
150.70 ± 11.90a |
- |
Doxorubicin |
535.00 ± 9.90c |
44.59 |
108.00±2.00d |
143.79 |
1022.20 ± 21.50d |
578.17 |
Doxorubicin + U. fasciata |
458.70 ± 7.40b |
-14.26 |
72.00±1.80c |
-33.33 |
679.30 ± 31.70c |
-33.55 |
Doxorubicin + AgNPs/U. fasciata |
361.70 ± 12.0a |
-32.39 |
66.30±1.70b |
-38.61 |
486.30 ± 25.40b |
-52.43 |
LSD at the 5% level | 13.96 | 2.44 | 30.68 | |||
F- probability | P<0.001 | P<0.001 | P<0.001 |
Data are expressed as Mean ± SE; Numbers of detected samples in each group is six; Means, which have different superscript symbol, are significantly different at p<0.05; Percentage changes were calculated by comparing doxorubicin-administered group with normal control and doxorubicin-administered groups treated with U. fasciata ethanolic extract and AgNPs/U. fasciata with doxorubicin-administered control groups.
Table 4: Effect of U. fasciata ethanolic extract and AgNPs / U. fasciata in kidney LPO product and SOD activity in doxorubicin-injected rats.
Parameters | LPO (nmole MDA/100mg tissue/hour) | % Change | SOD (mU/100mg tissue) | % Change |
Groups | ||||
Normal |
12.70 ± 1.30a |
- |
6.40 ± 0.20c |
- |
Doxorubicin |
23.70 ± 0.60c |
86.61 |
3.10 ± 0.30a |
-51.56 |
Doxorubicin + U. fasciata |
17.30 ± 0.20b |
-27.00 |
4.80 ± 0.10b |
54.84 |
Doxorubicin + AgNPs / U. fasciata |
13.70 ± 1.20a |
-42.19 |
4.90 ± 0.05b |
58.06 |
LSD at the 5% level | 1.19 | 0.25 | ||
F- probability | P<0.001 | P<0.001 |
Data are expressed as Mean ± SE; Numbers of detected samples in each group is six; Means, which have different superscript symbol, are significantly different at p<0.05; Percentage changes were calculated by comparing doxorubicin-administered group with normal control and doxorubicin-administered groups treated with U. fasciata ethanolic extract and AgNPs/U. fasciata with doxorubicin-administered control groups.
Table 5: Effect of U. fasciata ethanolic extract and AgNPs / U. fasciata on kidney GSH content and GPx and GST activities in doxorubicin-injected rats.
Parameters | GSH (nmole/100mg tissue) | % Change | GST (U/100mg tissue) | % Change | GPx (mU/100mg tissue) | % Change |
Groups | ||||||
Normal |
38.60 ± 1.20d |
- |
242.70 ± 9.20c |
- |
205.50 ± 1.40b |
- |
Doxorubicin |
23.30 ± 0.80a |
-39.64 |
104.00 ± 4.30a |
-57.15 |
166.50 ± 3.90a |
-18.98 |
Doxorubicin + U. fasciata |
28.10 ± 0.30c |
20.60 |
164.50 ± 1.10b |
58.17 |
173.30 ± 2.00a |
4.08 |
Doxorubicin + AgNPs/U. fasciata |
26.50 ± 0.60bc |
13.73 |
114.30 ± 4.20a |
9.90 |
173.80 ± 1.20a |
4.38 |
LSD at the 5% level | 1.09 | 7.13 | 3.79 | |||
F- probability | P<0.001 | P<0.001 | P<0.001 |
Data are expressed as Mean ± SE; Numbers of detected samples in each group is six; Means, which have different superscript symbol, are significantly different at p<0.05; Percentage changes were calculated by comparing doxorubicin-administered group with normal control and doxorubicin-administered groups treated with U. fasciata ethanolic extract and AgNPs/U. fasciata with doxorubicin-administered control groups.
The elevated serum CK-MB, AST and LDH activities reflect the increased leakage of these cytoplasmic enzymes from the damaged and necrotic cardiomyocytes to the blood (Farvin et al., 2004; Gürgün et al., 2008; Ahmed et al., 2017, 2019) as a result of doxorubicin cardiotoxicity. On the other hand, the doxorubicin-injected rats treated with U. fasciata and AgNPs/U. fasciata showed a significant decrease in serum CK-MB, AST and LDH activities. The treatment with AgNPs/U. fasciata was more effective than U. fasciata extract in preventing the elevation in serum CK-MB, AST and LDH activities in doxorubicin-injected rats. The decrease in these cardiac enzymes activities in serum as a result of treatment with U. fasciata and AgNPs/U. fasciata might be attributed to their capability to maintain membrane integrity, thereby restricting the leakage of these enzymes to blood. This attribution was supported by Rajadurai and Prince (2006), Zhou et al. (2006) and Zhou et al. (2008). Based on these results, it can be suggested that both U. fasciata and AgNPs/U. fasciata have potent cardiopreventive effects against doxorubicin-induced heart injury and AgNPs/U. fasciata was more potent.
Tables 4, 5, 6, and 7 showed the effect of U. fasciata and AgNPs/U. fasciata on the kidney and heart oxidative stress and antioxidant biomarkers in doxorubicin-injected rats. The treatment of doxorubicin-injected rats with U. fasciata and AgNPs/U. fasciata produced a remarkable increase of lowered GSH content and SOD, GST and GPx activities as compared to the corresponding doxorubicin-injected controls. In contrast, the elevated renal and cardiac LPO product in doxorubicin-injected rats significantly decreased (p<0.05) as a result of treatments with U. fasciata and AgNPs/U. fasciata which was more effective.
In our opinion, the enhancements in kidney and heart function and structural integrity in doxorubicin-administered rats treated with U. fasciata and AgNPs/U. fasciata may be attributed, at least in part, to the suppression oxidative stress and reinforcement of the antioxidant defense system in the kidney and heart. This suggestion is supported by the present results which revealed that U. fasciata extract and AgNPs/U. fasciata have detectable improvements effect on heart LPO product, GSH content, GST, GPx and SOD activities.
Effects on kidney histological changes
Photomicrographs of kidney histological sections of normal, doxorubicin-injected groups and doxorubicin-injected groups treated with U. fasciata and AgNPs/U. fasciata were shown in Figure 6 (Photomicrographs 6a-d).
Photomicrograph 6a showed normal histologic structure of glomeruli, proximal tubules and distal tubules. In doxorubicin-injected rats, severe glomerulonephrosis associated with severe focal inflammatory cells infiltration in the interstitial area were observed (Photomicrograph 6b). Doxorubicin-injected rats with U. fasciata showed mild degenerative changes of renal tubules and hypocellularity in the glomeruli (Photomicrograph 6c). Treatment of doxorubicn-injected rats with AgNPs/U. fasciata resulted in nearly normal histological architecture with mild inflammatory cells between renal tubules (Photomicrograph 6d).
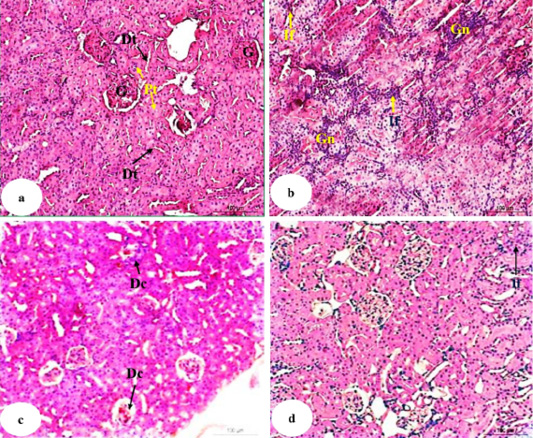
Figure 6: Photomicrographs of HandE stained kidney sections of normal (a), doxorubicin-administered group (b) and doxorubicin-administered groups treated with U. fasciata (c) and AgNPs/U. fasciata (d). G: glomeruli; Pt: proximal tubule; Dt: distal tubule; Gn: glomerulonephrosis; If: inflammatory cells infiltration; Dc: degenerative changes in glomeruli (with hypocellularity) and in tubules.

Figure 7: Photomicrographs of H and E stained heart sections of normal (7a), doxorubicin-injected group (7b) and doxorubicin-injected groups treated with U. fasciata (7c) and AgNPs / U. fasciata (7d). If: mononuclear inflammatory cells infiltration in the pericardium and between cardiomyocytes; and Nc: necrosis.
In the present study, the histolopathological changes due to doxorubicin-injection in the present study showed severe glomerulonephrosis and severe inflammatory cell infiltration in the interstitial area in accordance with
Table 6: Effect of U. fasciata ethanolic extract and AgNPs / U. fasciata on heart LPO product and SOD activity in doxorubicin-injected rats.
Parameters | LPO product (nmole MDA/100mg tissue/hour) | % Change | SOD (mU/100mg tissue) | % Change |
Groups | ||||
Normal |
29.70 ± 1.30a |
- |
5.30 ± 0.30ab |
- |
Doxorubicin |
52.80 ± 3.90c |
77.78 |
4.40 ± 0.10a |
-16.98 |
Doxorubicin + U. fasciata |
37.00 ± 0.90b |
-29.92 |
5.50 ± 0.20b |
25.00 |
Doxorubicin + AgNPs/U. fasciata |
33.00 ± 0.40ab |
-37.50 |
5.20 ± 0.40ab |
18.18 |
LSD at the 5% level | 2.74 | 0.42 | ||
F- probability | P<0.001 | P<0.001 |
Data are expressed as Mean ± SE; Numbers of detected samples in each group is six; Means, which have different superscript symbol, are significantly different at p<0.05; Percentage changes were calculated by comparing doxorubicin-administered group with normal control and doxorubicin-administered groups treated with U. fasciata ethanolic extract and AgNPs/U. fasciata with doxorubicin-administered control groups.
Table 7: Effect of U. fasciata ethanolic extract and AgNPs / U. fasciata on heart GSH content and GPx and GST activities in doxorubicin-injected rats.
Parameters | GSH (nmole/100mg tissue) | % Change | GST (U/100mg tissue) | % Change | GPx (mU/100mg tissue) | % Change |
Groups | ||||||
Normal |
15.00 ± 0.90bc |
- |
150.30 ± 2.00c |
- |
194.50 ± 3.30c |
- |
Doxorubicin |
10.60 ± 0.40a |
-29.33 |
102.50 ± 2.60a |
-31.80 |
181.80 ± 0.90a |
-6.53 |
Doxorubicin + U. fasciata |
13.70 ± 0.20b |
29.25 |
114.30 ± 2.60b |
11.51 |
186.70 ± 1.80ab |
2.70 |
Doxorubicin + AgNPs/U. fasciata |
14.90 ± 0.50bc |
40.57 |
108.10 ± 2.60ab |
5.46 |
195.30 ± 3.70c |
7.43 |
LSD at the 5% level | 0.93 | 3.13 | 3.54 | |||
F- probability | P<0.001 | P<0.001 | P<0.001 |
Data are expressed as Mean ± SE; Numbers of detected samples in each group is six; Means, which have different superscript symbol, are significantly different at p<0.05; Percentage changes were calculated by comparing doxorubicin-administered group with normal control and doxorubicin-administered groups treated with U. fasciata ethanolic extract and AgNPs/U. fasciata with doxorubicin-administered control groups.
Yilmaz et al. (2006) and Ahmed et al. (2017, 2019, 2020). The improvement in kidney histological architectures by treatment of doxorubicin-injected rats with U. fasciata and AgNPs/U. fasciata is concomitant with amelioration of serum biomarkers of kidney function, suppression of oxidative stress and enhancement of antioxidant defense system in the kidney. Thus, it can be suggested that the improvement in kidney histological integrity and function may be attributed, at least in part, to the attenuation of ROS production and reinforcement of antioxidant defense system mechanism in the kidney.
Effects on heart histological changes
Photomicrographs of heart histological sections of normal, doxorubicin-administered groups and doxorubicin-administered groups treated with U. fasciata and AgNPs/U. fasciata were shown in Figure 7 (Photomicrographs 7a-d).
In normal rats, no histopathological alterations were observed and normal histological structure of the myocardial muscle bundles were noticed (Photomicrograph 7a). In doxorubicin-administered rats, there was severe mononuclear inflammatory cells infiltration in the pericardium and between cardiomyocytes in association with necrotic changes (Photomicrograph 7b). These results are in agreement with Chen et al. (2017) and Ahmed et al. (2017, 2019, 2020).
After treatment of doxorubicin-administered rats with U. fasciata and AgNPs/U. fasciata, the heart sections showed nearly normal histological architecture and integrity (Photomicrographs 7c and 7d). These improvements in heart histological architecture and integrity were in concomitant with the ameliorations in the elevated serum biomarkers of heart function, suppression in lipid peroxidation and enhancement of the antioxidant defense system. Based on these evidences, it can be elucidated that the amendment of heart histological integrity and function may be ascribed, at least in part, to improvements in lipid peroxidation and the antioxidant defense system mechanisms in the heart.
CONCLUSION
The biosynthesis of silver nanoparticles using U. fasciata ethanolic extract as reducing agents is proved to be eco-friendly, efficient, rapid and simple method. TEM analysis showed that nanoparticles were produced exhibited large and small spherical particles and small percentages of rod with characteristic absorption peak at 200-400 nm and 600-800 nm in UV-Visible spectrum. In addition, FTIR spectrum revealed the presence of functional bioactive metabolites in algal extract which is responsible for the formation of AgNPs. The chemical constituents, alkaloids, fatty acids, terpernoids, phenolic compounds and aromatic compounds of studied algal extracts were identified by GC-MS; most of these constituents have reducing capability and may be responsible for formation of AgNPs. The results of this study also found that U. fasciata ethanolic extract and AgNPs/U. fasciata have effective preventive action against doxorubicin-induced renal and cardiac toxicities as confirmed by biochemical and histological findings. The AgNPs/U. fasciata was more potent in preventing the doxorubicin-induced kidney and heart toxicities. These preventive effects may be mediated, at least in part, via suppression of oxidative and enhancement of antioxidant defense mechanisms in both kidney and heart.
ACKNOWLEDGEMENTS
The authors thank Dr. El-Shaymaa El-Nahass, Associate Prof. of Histopathology, Pathology Department, Faculty of Veterinary Medicine, Beni-Suef University, Egypt and Prof. Dr. Rasha R. Ahmed, Professor of Molecular Cell Biology, Cell Biology, Histology and Genetics Division, Zoology Department, Faculty of Science, Beni-Suef University, Beni-Suef, Egypt for help in examining and determining the lesions in the kidney and heart histological stained sections.
AUTHOR CONTRIBUTION
Asmaa M. Mahmoud: Methodology, Formal analysis, Investigation, Software, Writing-Original Draft, Visualization, Data Curation; Basant M. Mohamed: Conceptualization, Methodology, Formal analysis, Investigation, Writing-Original Draft, Visualization, Validation; Ibraheem B. Mohammed: Conceptualization, Investigation, Writing-Reviewand Editing, Resources, Supervision; Hanan A. Soliman: Conceptualization, Investigation, Writing-Reviewand Editing, Visualization, Supervision; Osama M. Ahmed: Methodology, Formal analysis, Investigation, Writing-Original Draft, Visualization, Data Curation, Writing-Reviewand Editing, Supervision.
Conflict of interest
The authors have declared no conflict of interest.
REFERENCES