Advances in Animal and Veterinary Sciences
Review Article
The Potential of Pretreated Oil Palm Frond in Enhancing Rumen Degradability and Growth Performance: A Review
Nor Dini Rusli1,2, Ahmad Afifi Abdul Ghani1, Khairiyah Mat2, Mohd Termizi Yusof3, Mohd Zamri-Saad4, Hasliza Abu Hassim1,5*
1Department of Veterinary Preclinical Sciences, Faculty of Veterinary Medicine, Universiti Putra Malaysia, 43400 UPM Serdang, Selangor, Malaysia; 2Department of Agriculture Science, Faculty of Agro-Based Industry, Universiti Malaysia Kelantan, Jeli Campus, 17600 Jeli, Kelantan, Malaysia; 3Department of Microbiology, Faculty of Biotechnology and Biomolecular Sciences, Universiti Putra Malaysia, 43400 Serdang, Selangor, Malaysia; 4Department of Veterinary Laboratory Diagnosis, Faculty of Veterinary Medicine, Universiti Putra Malaysia, 43400 Serdang, Selangor, Malaysia; 5Laboratory of Sustainable Animal Production and Biodiversity, Institute of Tropical Agriculture and Food Security, Universiti Putra Malaysia, 43400 Serdang, Selangor, Malaysia.
Abstract | A million tons of agricultural by-products are produced each year. Many farmers in tropical countries used this by-product as the main feed sources for livestock feeding. Nonetheless, most of the agricultural by-products have poor nutritional value, primarily due to high crude fibre content, low crude protein, total fat, energy, mineral and vitamin contents. Similarly, oil palm frond (OPF) contains high lignocellulosic content with low nutritional value which can be important restrictions on the use of OPF as livestock feed. The chemical composition showed that OPF was composed of 70% fibre and 22% soluble carbohydrates on a dry matter (DM) basis. This problem has resulted in reduced animal production. Various approaches were investigated to enhance these feeds, including physical, chemical and biological pretreatments. Biological pretreatment may be amongst the most practical because it is safe and effective in decreasing content of fibre fractions with increased content of crude protein. Further research is required to investigate the biological pretreatment with enzyme extract from white rot fungi to reduce dry matter losses and shorten duration of incubation time. This review highlights the lignocellulose content of the agricultural by-product especially OPF, the shortcomings of rumen microbes in degrading the agricultural by-product and pretreatment strategies for OPF. The review also includes the effect of pretreated OPF on rumen degradability and growth performance. This knowledge can be used in upgrading the OPF as ruminant feed as the basis for future study.
Keywords | Oil palm frond (OPF), Agricultural by-product, Pretreatments, Rumen degradability, Growth performance
Received | December 12, 2020; Accepted | January 11, 2021; Published | April 15, 2021
*Correspondence | Hasliza Abu Hassim, Department of Veterinary Preclinical Sciences, Faculty of Veterinary Medicine, Universiti Putra Malaysia, 43400 UPM Serdang, Selangor, Malaysia; Email: haslizaabu@upm.edu.my
Citation | Rusli ND, Ghani AAA, Mat K, Yusof MT, Zamri-Saad M, Hassim HA (2021). The potential of pretreated oil palm frond in enhancing rumen degradability and growth performance: a review. Adv. Anim. Vet. Sci. 9(6): 811-822.
DOI | http://dx.doi.org/10.17582/journal.aavs/2021/9.6.811.822
ISSN (Online) | 2307-8316; ISSN (Print) | 2309-3331
Copyright © 2021 Hassim et al. This is an open access article distributed under the Creative Commons Attribution License, which permits unrestricted use, distribution, and reproduction in any medium, provided the original work is properly cited.
INTRODUCTION
The increases of human population, urbanisation and incomes worldwide, over the last decades have influenced greatly the demands for meat and milk products (Fróna et al., 2019). With an estimated 60% increase in demand for food by 2050 (FAO, 2013), there could be competition between feed and food, therefore it is imperative that ruminant feed has to rely on feed materials not utilized by humans to avoid competition between animal and human nutrition. This has become a growing concern and has driven researchers to investigate the potential of agricultural by-product as ruminant feed and improve the constraints on the use of some feed resources. The use of these agricultural by-products in most emerging and developing countries is actually aimed at reducing feed costs as well as environmental pollution (Dahlan et al., 2000). Besides, the use of agricultural by-products can minimise the expensive imported feedstuff as the local by-products have the potential to substitute as feedstuff component. This will be leading to the reduction of the cost for livestock production.
In Malaysia, the oil palm plantation areas are 5.23 million hectares as of 2013, representing 60% of the total agricultural land (Kamalrudin & Abdullah, 2014). Hence, abundant oil palm by-products had been generated each year in the form of oil palm trunk (OPT), empty fruit bunches (EFB), oil palm frond (OPF), palm oil decanter and palm kernel cake (PKC). These by-products act as an alternative to ruminant diets as it helps to solve problem related to lack of grazing pasture (Abubakr et al., 2015). The OPF was reported to be the major by-product of palm oil production which is 47% out of the total oil palm waste (Nordin et al., 2017). They are being generated from harvesting and pruning, which are also left to decay for nutrient conservation in the soil (Wan Zahari et al., 2003). The OPF is the most generated oil palm by-product, which is amounted 56 million tonnes annually during replanting (Yusof et al., 2019). However, there is a higher percentage of crude protein (CP) and ether extract (EE) on the leaves than the petioles (Mohamed et al., 2012). The OPF is commonly being fed to beef and dairy animals at 50% and 30% of inclusion level, respectively (Wan Zahari et al., 2003). Most local farmers in Malaysia extensively chopped the OPF and combined with other feedstuff as total mixed rations (Ng et al., 2011). Adding OPF to beef and dairy cattle diets could help live weight gain between 0.6 and 0.8 kg per day, and milk yields of around 22 litres per day (Wan Zahari et al., 2003). Despite previous reports of OPF as a promising roughage source for ruminants, there is still limited use of OPF for ruminant feeding. Furthermore, OPF can not be used exclusively as animal feed because of its poor nutritional values with low metabolizable energy of 4.9 to 6.5 MJ / kg dry matter (DM; Dahlan et al., 2000). Oil palm frond’s lignin content is also high at 205g/kg DM on average (Nordin et al., 2017) and can affect OPF intake and digestibility. Lignin, the most recalcitrant part of the cell wall of plants, serves as a barrier and restricts rumen microorganisms to degrade OPF. In addition, these rumen microorganisms are not prepared for lignin degradation because they do not generate enzymes with ligninolytic activity (Pollegioni et al., 2015), resulting in a reduced degradability (often less than 50%) of the original material, leading to a low metabolizable energy (ME) content (< 7.5 MJ/kg DM). In reality, feeding ruminants with only OPF without any additional feed to cover the requirements of all nutritional ruminal microorganisms will result in poor degradability that is further reflected in animal performance.
Efforts are therefore being made to establish pretreatment methods of OPF to improve the nutritional quality of OPF as a feed required for the production of new feeding and forage strategies and to avoid the shortage of healthy feed (Rusli et al., 2019). One of the latest developments in the management of oil palm waste is the improvement in the nutritional value of OPF to maximise the ability of locally available feedstuffs and to enhance their use as an animal feed resource.
This review addresses the difficulties of rumen microbes in degrading the agricultural by-products, as well as various pretreatment approaches to resolve recalcitrance of agricultural by-products and boost rumen degradability and growth performances of ruminants.
Plant Cell Wall Of Oil Palm Frond
Plant cell wall mainly consists of complex polysaccharides (sugar-based polymers) molecules such as cellulose, hemicellulose and pectin. In between the cellulose, hemicellulose and pectin components, lignin acts as the structural support of the cell wall by crosslinking different polysaccharides (Figure 1). Plant cell wall refers to lignocellulosic biomass which also consists of water, wood, small amounts of protein and other plant substances (Zeng et al., 2017). Hydrogen bonds hold all the compositions cellulose, hemicellulose and lignin strongly. On top off that, chemical bonding also exists between hemicellulose and lignin, making the lignin contains fewer amounts of carbohydrates.
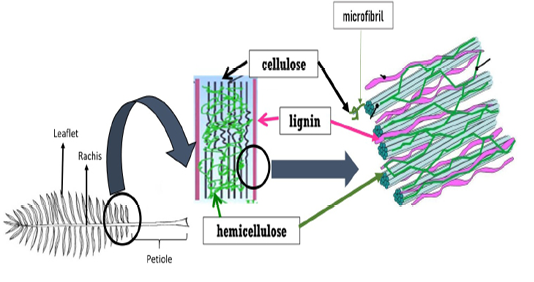
Figure 1: The structure of lignocellulosic biomass
Cellulose is a major component of plant cell walls, providing cell stiffness and strength. Cellulose is a highly insoluble organic compound where D-glucose subunits are joined to each other and linked by β-1,4 glycosidic bonds forming the dimer cellobiose. Cellulose consists of crystalline and noncrystalline structures. In its crystalline form, a long chain of glucose molecules are bound together in the microfibrils by hydrogen bonds. The cellulose microfibrils are extensively cross-linked by hemicellulose-lignin matrix and are often tethered in the form of bundles or macrofibrils. Meanwhile, the noncrystalline portion is often called amorphous cellulose. Crystalline cellulose may be degraded at a slower rate than amorphous region by which this characteristic limits ruminal microbial degradation. Being the second most abundant polysaccharide in plant cell wall, hemicellulose composed of D-xylose, D-mannose, D-galactose, D-glucose, L-arabinose, 4-0-methyl-glucuronic, D-galacturonic and D-glucuronic acids. While cellulose polymers can form ordered crystalline structure which is strong and resistant to hydrolysis, hemicellulose has an amorphous structure with a lower molecular weight than cellulose. Importantly, hemicellulose is greatly branched with the presence of acetyl groups but lacking the crystalline structure (Zhou et al., 2017).
Lignin comprises 15-25% of the cellulosic biomass (Zhou et al., 2017) and is linked to both components of plant cell wall; hemicellulose and cellulose by esterified bonds. Lignin is a complex natural polymer that provides strength to the plant. Since lignin is the most recalcitrant component of plant cell wall and a highly insoluble, the higher the content of lignin, the lower the carbohydrate degradation. Lignin acts as binder that is an impenetrable barrier between cells affecting the bioavailability of the cellulose and hemicellulose for microbial use. Consequently, this would affect the nutritive value of a plant material for animals, affect the digestibility and inhibit the rate and degree of microbial degradation.
Moreover, the cell wall of OPF also consists of ash, glucose and xylose. Previous study reported that OPF contains higher hemicellulose content than pineapple, banana, and even soft and hardwood fibres (Abdul Khalil et al., 2006). The existence of cross-linkages between cellulose and lignin hemicellulose via ester and ether linkages resulted in OPF resistance.
Limitation of Rumen Microbes’ Ability to Degrade Plant Cell Wall
The feeding value of agricultural by-product particularly roughage, is limited due to less nitrogen content and high in lignocellulosic compounds, which therefore in turn producing low fermentable carbohydrates. The cell wall content of roughage and its nature of lignification are amongst the key factors which rule the degradability and the passage rate of forage. Meanwhile the nature of the microbial enzymes and accessibility of the dietary polysaccharides by these enzymes are also the other factors that govern the rate and extent of the complex dietary polysaccharides (eg. cellulose and hemicellulose).
The main interest of the most studies was the lignin because it is the major culprit in restricting the use of local agricultural by-product such as OPF as ruminant feed. They made up the lignocellulosic biomass, along with the hemicellulose material. Previous reports demonstrated that the ester bonds between lignin, hemicellulose and cellulose hamper the degradation of wheat straws (van Kuijk et al., 2017) rice straw (Dinh Vu et al., 2017) and OPF (Rasli et al., 2017). The lignin consists primarily of three aromatic alcohol precursors that are coniferyl alcohol, sinapyl, and p-coumaryl. These alcohol are called guaiacyl – (G), syringyl- (S) and p-hydroxyphenyl (H) lignin subunits respectively. The ratio of these subunits differs by species of plants which give them different composition of lignin. The oxidative coupling of aromatic alcohols provide a complex structure of lignin. By linking to both hemicellulose and cellulose, lignin acts as a barrier to any solution of enzyme preventing degradation which can prevent the enzymatic degradation. Specifically, lignin contributes to the inhibition of enzymatic hydrolysis via non-specific or non-productive binding to the enzyme. Besides, lignin in lignocellulosic biomass retards the physical barrier which blocks enzyme access and microbial colonisation of fermentable cellulose and hemicellulose (Li et al., 2016).
Ruminants’ ability to digest plant material is attributed by their symbiotic relationship with rumen microbes. The bacterial species play an important role in determining the rate of degradation of the feed for microbial protein and fermentation end-product such as volatile fatty acid production (Wang et al., 2020). The presence of lignin in lignocellulosic materials can limit the process of rumen bacteria adhesion to plant tissue for subsequent cell wall degradation. The adhesion process of rumen bacteria can be described by dividing the process into four steps (Nunes & Kumar, 2018). The first step is conveying of non-motile bacteria to the substrate. Next, the initial non-specific adhesion of bacteria to unprotected surface of the substrate that is mediated by bacterial glucocalyx constitutive elements. Then, there is the basic adhesion caused by adhesion or formation of ligands. Several bacterial organels that include cellulosome complexes, fimbriae formation, glycosylated epitopes of cellulose-binding protein or glocucalyx, and enzyme-binding cellulose domain facilitate adhesion. The final step is bacterial proliferation on the digestible substrate. The consequence of low digestibility of agricultural by-products due to the lignin restriction can cause low production of VFA, which translates into less energy supply to the animal, adversely affecting its output in the production of growth, meat and milk.
Furthermore, different plant fractions of agricultural by-products give different ruminal degradability values. For instance, leaflet who is most nutritious fraction of OPF, is slightly more digestible than the petiole as the degradability of both leaflet and petiole are 435 g/kg DM and 391 g/kg DM, respectively (Dahlan et al., 2000). The metabolisable energy (ME) values of different inclusions of OPF were reported to be 4.9 MJ/kg DM for OPF 200 and OPF 300 incubations and 5.8 MJ/kg DM OPF for OPF 100 incubation (Hassim et al., 2010). These results indicate that OPF digestion rate was impaired with higher proportions of OPF and suggest that there is dissimilarity amongst batches and/or the origin of the OPF product which can influence the extent of its rumen degradability and apparent digestibility. Meanwhile, the stem of rice straw contains less ash and more NDF, generated a higher in vitro DM degradability (610 g/kg DM; Sarnklong et al., 2010) compared to the leave part of rice straw (510 g/kg DM).
Strategies To Improve Ruminal Degradability Of Oil Palm Frond
The basic key in increasing the ruminal degradability following the utilisation of agricultural by-product to improve the access of rumen microbes to cellulose and hemicellulose (Figure 2). Pretreatment technology is a vital step in facilitating the conversion of lignocellulosic biomass into fermentable sugars, aiming to increase microbial enzyme accessibility to biomass and consequently transform the carbohydrate polymers into fermentable sugars (Mosier et al., 2005). The main goal of pretreatment is particularly to loosen the complex structure of lignin and interrupt the crystalline structure of cellulose but minimise cellulose degradation and formation of inhibitory compound like phenolic who is crosslinking with hemicelluloses. Therefore, in order to overcome the challenge to improve the degradability in agricultural by-product, the researches investigated few strategies which include pretreatements of agricultural by-products. Pretreatment strategy could improve the by-products’ nutritive value, enhancing feed intake and digestibility by breaking the binding of lignin who is bound together with hemicellulose and cellulose. This step allows the rumen microbes to access to these fibres and degrade them (Figure 2).
To date, numerous physical, chemical and biological pretreatments have been developed to upgrade the agricultural by-products through an improvement in hydrolysis of lignocellulosic bonds (Figure 3). The choice of the pretreatment strategies for breaking the lignocellulose structure of agricultural by-product depends on the economic and environmental impact. In the following sections, the most common pretreatments of agricultural by-products are described with its effects on the ruminal degradability and animal performance.
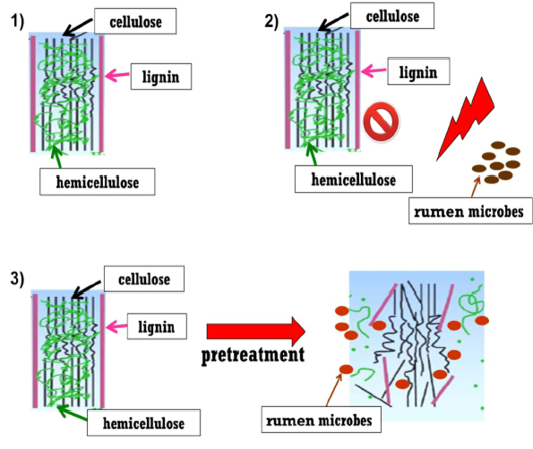
Figure 2: Steps of pretreatment strategy.
Effect Of Physical Pretreatment Of Oil Palm Frond On Rumen Degradability And Growth Performance Of Ruminants
Physical or mechanical pretreatment involves breakdown of particle size and reduction of crystallinity by choping, grinding, pressing and milling. In many practices, physical pretreatment is usually applied as the first method before the biomass is pretreated with other pretreatment strategy. Table 1 presents an overview of the previous studies who reported about physical pretratment of agriculture by-products on rumen degradability. Physical pretreatment by chopping was shown to increse fermentation quality of rice straw silage compared with the non-chopped silage (Zhang et al., 2010). Increased particle size and physical effective fibre increased rumination time, chewing activity and ruminal pH, which provide a good medium for the growth of rumen microbes; but did not show any influence on feed intake (Zhao et al., 2009).
In Malaysia, whole OPF which include petiole and leaflet are commonly chopped to 2 to 3 cm lengths and fed either
Table 1: Overview of the physical pretratment of different agricultural by-products
Physical pretreatments | Agricultural by-products | Assessment of Parameters | Results/Relative change (%) compared to non-treated samples | References |
Chopping | Rice straw (silage) |
In vitro, dairy cows, VFA concentration |
+63 |
Zhang et al., 2010 |
Rice straw
|
In vivo, goats, VFA concentration |
No change |
Zhao et al., 2009 |
|
Dry matter intake | No change | |||
Starch digestibility | +10 | |||
Pelletising |
Rice straw |
In vivo, ARDC based on total SCFA production |
+24 | Ware and Zinn, 2005 |
Propionate concentration | +47 | |||
Ruminal pH | -9 | |||
OPF |
In vivo, goat, apparent DM digestibility |
+13 | Dahlan et al., 2000 | |
In vivo, goat, apparent CP digestibility |
+58 | |||
In vivo, goat, apparent ADF digestibility |
+52 | |||
Grinding | Rice straw |
In vivo, cattle, apparent digestibility of DM, CP, NDF and ADF |
No change |
Yuangklang et al., 2010 |
Steam explosion (120◦C) |
Rice straw |
In vitro, 96h, DM degradability |
+24 | Chaji et al., 2010 |
In vitro, 96h, NDFdegradability |
+26 | |||
Steam explosion (198◦C) |
Wheat straw |
In vivo, ram, apparent DM digestibility |
+17 | Viola et al., 2008 |
Pressing | OPF |
In vitro, VFA concentration |
+3.35 |
Rusli et al., 2019 |
Table 2: Overview of the chemical pretratment of different agricultural by-products
Chemical pretreatments | Agricultural by-products | Assessment of Parameters | Results/Relative change (%) compared to non-treated samples | References |
NaOH | Rice straw |
In sacco, DM degradability |
+16 | Suksombat, 2004 |
Barley straw |
In vivo, sheep, apparent DM digestibility |
+28 | Arisoy, 1998 | |
Sugarcane bagasse |
In sacco, DM degradability |
+35 | Suksombat, 2004 | |
OPF | Lignocellulose composition (lignin) | -30 | Sukri et al., 2014 | |
Urea |
Rice straw |
In vivo, cow, apparent DM digestibility |
+22 | Wanapat et al., 2009 |
In vivo, cow, apparent NDF digestibility |
+23 | |||
In vivo, cow, apparent ADF digestibility |
+22 | |||
Wheat straw |
In vitro, DM degradability |
+26 |
Kraidees, 2005 |
|
In vitro, NDF degradability |
+41 | |||
Sugarcane bagasse |
In sacco, DM degradability |
-6 | Suksombat, 2004 | |
Ammonia | Sorghum stover |
In vivo, sheep, apparent OM digestibility |
+11 | Salem et al., 1994 |
In vivo, sheep, apparent NDF digestibility |
+27 |
fresh, dried, pelleted or ensiled in combination with other ingredients as total mixed rations. Pelletising is one of the effective ways that has been used to increase the feeding value of OPF (Dahlan et al., 2000). Pelletising is the process of compacting the feed material into a different shape of pellet. The process involves few steps including drying, milling, conditioning, actual pelletising and cooling of the biomass. Dahlan et al. (2000) observed that pelletising OPF increased its nutrient digestibility on the goats and produced high total VFA concentration. Nevertheless, pelletising process requires high energy which makes the technique not suitable for small-scale farms. Steam explosion is well-known for pretreatment of lignocellulosic biomass. Steam explosion is used by treating the biomass with high pressure saturated steam at a temperature of 160-260°C (corresponding pressure 0.69-4.83 MPa) before the pressure is lowered to the atmospheric value, which makes the biomass undergo an explosive decompression. Steam explosion pretreatment is most effective and commonly used for agicultural by-product, for example rice straw and wheat straw (Chaji et al., 2010; Viola et al., 2008). It was shown that steam explosion pretreatment increased the in vitro degradability of rice straw and in vivo apparent digestibility of wheat straw. It only requires the low energy compared to mechanical comminution. The process causes degradation of lignin-hemicellulose bound, thus increasing the potential of ruminal microbial enzymes to hydrolyze cellulose. When steam-treated OPF supplemented with up to 16 g urea/kg OPF was administered to lambs, DM intake, OM intake and digestibility of DM showed substantial linear increases (P < 0.001) (Bengaly et al., 2010). Paengkoum et al (2006) found that feeding steam-treated OPF with high energy levels (16.20-16.24 MJ/kg) could enhance the use of urea supplementation (optimal 3%) in Saanen goat feed, which revealed positive results of animal performance in conjunction with improved ruminal fermentation, microbial yield and N balance.
Recently, pressing method of OPF using conventional sugarcane pressing machine was used to obtain 50 wt% of OPF juice (Zahari et al., 2012). The OPF juice released a substantial amount of sugars which was 76.09 g/l, whereby glucose as a major sugar component (53.95 g/l), accounting 60 to 70% of total free sugar (Zahari et al., 2012). The other sugars present in addition to glucose were sucrose (20.46 g/l) and fructose (1.68 g/l). Indeed, this could provide a favourable environment for the microbial fermentation in the rumen. Furthermore, the process of pressing OPF is considered as cost practical, easily operated, contain no impurities, low risk of health and safety and indeed produce high yields of OPF juice. Our recent study also reported the use of sugarcane pressing machine to physically press the OPF to obtain the OPF pressed fibre for chemical analysis and in vitro ruminal degradability (Rusli et al., 2019).
Effect Of Chemical Pretreatment Of Oil Palm Frond On Rumen Degradability And Growth Performance Of Ruminants
Several chemical pretreatments which usually involve the use of alkaline, acidic or oxidative agents have been conducted in improving the nutritional quality of agricultural by-products (Sarnklong et al., 2010). Alkaline solution is the most widely used chemical agent in chemical pretreatment especially sodium hydroxide (NaOH), ammonia (NH3) and urea. This pretreatment needs low temperature and pressure management, compared to other pretreatment strategies (Baruah et al., 2018). These alkali pretreatments have been shown to cause the degradation of ester and glycosidic side chains, resulted in enzymatic saccharification of cellulose and hemicellulose during ruminal fermentation (Kucharska et al., 2018).
An overview of the effect of chemical pretreatment of agricultural by-products on different parameters is presented in Table 2. Sodium hydroxide treatment affects the constitution of natural lignin, facilitating the activity of microbial enzymes by improving the substrate-microbial enzyme contact and hence, increasing fibre digestion. Chemical pretreatment of bagasse and rice straw with NaOH was reported to increase feed intake, ruminal degradability and digestibility in ruminants (Suksombat, 2004). Several reports demonstrated that NaOH pretreatment was effective in increasing DM degradability of rice straw, barley straw and sugarcane bagasse (Table 2). A recent study reported that pretreated OPF with NaOH reduced the lignin content from 35.79% to 25.48% but increased and produced the highest sugar production at 2.93 mg/mL (Sukri et al., 2014). This phenomenon suggests that delignification assisted the production of reducing sugar which was attributed by the increased enzymatic digestibility of cellulose and hemicellulose due to the increased holocellulose-lignin bond disruption.
Kawamoto et al. (2001) stated that, compared to OPF pellets and silage, NaOH treated OPF had higher DM digestibility and digestible DM intake (DMI) in cattle, although it had low palatability. Similarly, Ooi et al. (2017) demonstrated that treatment with NaOH was adversely affected to palatability of OPF in cattle.Based on animal feeding trials, it was suggested that the low quality of the fermentation may reduce the palatability of OPF silage treated with NaOH. The inclusion of OPF treated alkali in the concentrate or roughage portion of the complete feed has been shown to be beneficial in promoting growth efficiency and enhancing digestibility, N-balance and beef cattle rumen fermentation. However, the use of NaOH in chemical pretreatment of agricultural by-products is limited due to its high price, hence it is not economically feasible in some countries especially developing and transformation countries (Ooi et al., 2017). However because the chemical is caustic and hazardous, it is necessary to develop a safer treatment procedure and a more cost-effective method.
Urea and ammonia are also widely used for chemical pretreatment of agricultural by-products. Wanapat et al. (2009) showed urea treatment could increase the apparent digestibility of rice straw by making cellulose and hemicellulose available to microbial enzymes in the rumen. Similarly, chemical pretreatment using urea also increased the
Table 3: Overview of the biological pretratment of different agricultural by-products
Biological pretreatments | Agricultural by-products | Assessment of Parameters | Results/Relative change (%) compared to non-treated samples | References |
Ceriporiopsis subvermispora |
Sugarcane bagasse |
In vitro, OM degradability |
+35 | Okano et al., 2006 |
In vitro, NDF degradability |
+27 | |||
Pleurotus eryngii |
In vitro, OM degradability |
+5 |
Okano et al.,, 2007 |
|
In vitro, NDF degradability |
-2 | |||
Pleurotus pulmonarius |
Maize straw |
In vitro, OM degradability |
+16 | Akinfemi et al., 2009 |
In vitro, 24h, gas production |
+27 | |||
C.subvermispora, Lentinula edodes, Pleurotus eryngii and Pleurotus ostreatus |
Oil palm frond |
In vitro gas production |
+68 | Tuyen et al., 2013 |
C.subvermispora; Lentinula edodes | Sugarcane bagasse |
In vitro gas production |
+43 | |
Pleurotus eryngii; Pleurotus ostreatus |
Sugarcane bagasse |
In vitro gas production |
-12 | |
Lentinussajor-caju | Oil palm frond | DM, OM, Ash digestibility | No change |
Chanjula et al., 2017 |
Ganoderma lucidum
Lentinula edodes
Phlebia brevispora
Phanerochaete chrysosporium
Trametes versicolor |
Oil palm frond |
In vitro, Total VFA, 9 weeks |
+9 |
Rahman et al.,2011 |
In vitro, ARDC, 9 weeks |
+10 | |||
In vitro, Total VFA, 9 weeks |
+38 | |||
In vitro, ARDC, 9 weeks |
+30 | |||
In vitro, Total VFA, 9 weeks |
+38 | |||
In vitro, ARDC, 9 weeks |
+28 | |||
Oil palm frond | Lignin content |
-15
-9 |
Hermiati et al., 2013 |
|
Pleurotus ostreatus |
Corn straw | Average Daily Gain (ADG) of lambs | +17.5 | Ramirez-Bribiesca et al., 2010 |
Trichoderma ressi | Corn stalk | DM intake | +3 |
Omer et al., 2012
|
ADG of lambs | +28 | |||
Cellulase and xylanase |
Corn stover
|
Organic matter digestibility | +10 |
Vallejo et al., 2016
|
Metabolisable energy | +10 | |||
VFA concentration | +16 | |||
Maize stover |
In vitro DM digestibility |
+38 | Bhasker et al., 2013 | |
Rice straw | DM digestibility | +14 |
Mao et al., 2013! |
|
NDF digestibility | +13 |
degradability of wheat straw and sugarcane bagasse (Kraidees, 2005; Suksombat, 2004). Urea is considered safe and cost effective as compared with NaOH pretreatment which is corrosive and dangerous. Meanwhile, ammonia hydrolyses the lignocellulosic bonds of plant carbohydrates making cellulose and hemicellulose more accessible to microorganisms in the rumen, increasing ruminal degradability of lignocellulosic material. Salem et al. (1994) reported that ammonia pretreated sorghum stover increased OM and NDF digestibility in sheep. A feeding trial conducted by Muthalib and Afdal (2018) showed that 50% ammonia-treated OPF appeared to increase carcass weight, rib-eye muscle area and Bali bulls’ meat chemical content. There are few factors that should be considered to ensure the effectiveness of urea pretreatment such as the urea level, moisture content, duration of the treatment and type and quality of agricultural by-products. One of drawbacks of urea pretreated feed to ruminant is excessive ammonia production in the rumen which can lead to toxicity.
Effect Of Biological Pretreatment Of Oil Palm Frond On Rumen Degradability On Rumen Degradability And Growth Performance Of Ruminants
Biological pretreatment provides a natural and safe way of treating and effectively removing lignin to enhance digestibility of the lignocellulosic biomass. The biological pretreatment of lignocellulosic biomass is usually applied microorganism such as brown, white and soft rot fungi to attack degrade lignin and hemicellulose. Apart from low energy requirement and mild operation conditions, biological pretreatment offers a green, safe and cost effective approach, comparing with physical and chemical pretreatments. An overview of the effect of biological pretreatment of OPF on rumen degradability and growth performance, such as fungi and commercial fibrolytic enzymes, are shown in Table 3.
There are lots of studies about the application of several white rot fungi (WRF), basidiomycetes in pretreating the agricultural by-products. They are well known for their capability to secrete extracellular ligninolytic enzymes breakdown the lignocellulosic bonds. Indeed, WRF were reported to be the most effective basidiomycetes for biological pretreatment of agricultural by-products such as sugarcane bagasse (Okano et al., 2006; Tuyen et al., 2013), maize straw (Akinfemi et al., 2009), OPF (Rahman et al., 2011; Tuyen et al., 2013; Chanjula et al., 2017). The WRF ‘s selective property of decomposing lignin, cellulose , and hemicellulose into simple carbohydrates as a carbon and energy source is mainly due to the extracellular lignolytic enzymes include lignin peroxidase (LiP), manganese-dependent peroxidase (MnP) and laccase (Vrsanska et al., 2016). A number of studies have demonstrated several WRF strains from the genera Ceriporiopsis, Pleurotus, Lentinula and Ganoderma to selectively degrade lignin of agricultural by-products by improving their ruminal degradability (Table 3). However, the length of the OPF colonization by WRF depends on the fungal species, fungal strain, substrate and culture conditions. Different strains of the same fungal species yields different ability of degrading the lignin-hemicellulose-cellulose ratio from the same substrate (Nadir et al., 2019). Hermiati et al. (2013) showed that pretreatment of OPF fibre using two species of WRF Phanerochaete chrysosporium (P. chrysosporium) and Trametes versicolor (T. versicolor) for 4 weeks reduced lignin content of OPF but P. chrysosporium was able to also decrease hemicellulose content. T. versicolor was found to be more effective than P. chrysosporium in producing the highest reducing sugar yield (12.61% of dry biomass). Biological treatments of OPF by solid state and submerged fermentations in the presence of ten WRF such as Bjerkandera adusta, Ceriporiopsis subvermispora, Ganoderma lucidum, Lentinula edodes, Phanerochaete chrysosporium, Phlebia brevispora, Schizophyllum commune, Pleurotus eryngii, Pleurotus ostreatus, Trametes versicolor were evaluated following 3 weeks and 9 weeks of colonization on the ruminal degradability (Rahman et al., 2011). The most promising effect of C. subvermispora (3 wks) and L. edodes and P. brevispora (9 wks) for biological pretreatment of OPF were reported but the relatively high biomass losses during fungi colonization should be taken into account in biological pretreatment. However the approach of WRF colonisation is still time consuming and causing DM losses due to fungal metabolism. The breakdown by lignocellulosic biomass by WRF involves long-chain polysachharides, mainly cellulose and hemicellulose as a source of carbon and energy while degrading the lignin. Therefore, a proper method to preferentially remove lignin, but not polysaccharides (cellulose and hemicellulose), are needed if biological pretreatment with WRF is to be successful. Study has shown that this limitation can be resolved by minimizing the DM loss in wheat straw when the pretreatment used enzyme extract from WRF as compared with the WRF mass (Rodrigues et al., 2008).
Several attempts have been made previously in using exogenous fibrolytic enzymes to improve the quality of forage and the efficiency of animal performance (Peters et al., 2015). For example, the organic matter digestibility, metabolisable energy and VFA were increased following the pretreatment of corn stover with cellulase and xylanase (Vallejo et al., 2016). Similarly, Togtokhbayar et al. (2015) reported that wheat straw pretreated with xylanase improved gas production and VFA. Such enzymes have a cellulolytic and hemicellulolytic capacity to target crop residue lignocellulose structure to improve their feeding efficiency (Abdel-Aziz et al., 2015). Enzyme supplementation to ration is followed by increased intake of feed, which may be attributed in part to improve dietary palatability attributed to sugars produced by pre-emptive hydrolysis of fibre. Nonetheless, effects of the post-ingestive enzyme, such as increased digestion rate and degree of digestion, can increase hydrolysis in the rumen to decrease gut filling and increase feed intake. Regarding lactating dairy cows and growing cattle, positive effects of introducing exogenous enzymes to the ruminant diets have been identified. Dairy cows fed drilling treated with a fibrolytic enzyme additive consumed more feed and produced 5-25% more milk, improved dairy cows’ transitional energy balance and increased milk production in small ruminants (Peters et al., 2015).
Several studies have examined the impact of biologically pretreated OPF feeding on the growth performance of various species of ruminants under a range of conditions. Hamchara et al. (2018) discovered that OPF feeding which was treated with different levels of Lentinus sajor-caju could promote digestibility and nitrogen (N) retention in crossbred male goats, suggesting that the application of fungus-treated OPF provides an alternative forage feedstuff that constitutes up to 100% OPF. This finding is consistent with the results of Febrina et al. (2017), who showed that OPF fermented with Phanerochaete chrysosporium plus minerals was the best diet for goats, as indicated by body weight gain and low feed conversion (S, P and Mg). Mineral inclusion may promote the growth of P. chrysosporium and enhance the activity of ligninolytic enzymes in fungi. However in finishing crossbred male goats (Thai Native Anglo Nubian) fed total mixed rations (TMR) containing 30% L. sajor-caju treated OPF, Chanjula et al. (2017) observed no significant difference in feed intake, efficiency, carcass traits or meat quality.
While biological pretreatment improves the fermentation quality of agricultural by-products as well as improve feed intake and digestibility of the animals, further investigation is required in the form of TMR containing agricultural by-product dry content following the enzyme extract from the WRF. Application on a farm scale, however, requires further research considering cost development as well as safety and health of the animals. In Malaysia, significant attention has been paid to enhancing the nutritional value of oil palm by-products especially OPF by processing and supplementation. Nonetheless, relatively very few feeding trials were performed especially in Malaysia, in order to delineate the relationships between production, nutrition and animal performance in the sense of maximizing the pretreated OPF. Furthermore, understanding and overcoming the barriers to microbial fermentation of OPF cell wall by, for example, biological pretreatment with enzyme extract from OPF may greatly improve OPF feeding value.
CONCLUSION
Oil palm frond is known as the main source of biomass that has been used as a substitute for tropical grasses as feedstuffs for ruminants in the tropics. Because of poor nutritive values with low metabolisable energy and high lignocellulosic content, its use as a livestock feed is limited. The pretreatment method of OPF is therefore an extremely important step in the conversion of OPF into value-added feed. The effects of various pretreatment approaches on OPF, nutritional value, and chemical composition have been thoroughly studied. Analysis of different pretreatment methods leads us to the conclusion that each procedure has shown promising results in relation to the breakdown and alteration of the lignocellulosic structure in OPF to make lignocellulosic substrates readily accessible by rumen microbes to enzymatic hydrolysis, thus growing the apparent DM and organic matter degradability of in vitro and in vivo rumen, and improving growth efficiency. Nevertheless, biological pretreatment is considered a preferable choice among the pretreatments due to its practicality, environmentally sustainable and safer approach to raising the nutritional value of OPF as animal feed. However, combination pretreatment can be considered to be used for shortening the delignification cycle in the future. Hence, more work is required to improve combined pretreatment to its maximum potential to enhance the degradability of rumen and animal performance.
ACKNOWLEDGMENTS
This research was supported by the Ministry of Higher Education, Malaysia under the Fundamental Research Grant Scheme (FRGS/1/2017/WAB01/UPM/02/18). The authors thank all staffs and postgraduate students from Feed Analysis Lab, Amirul Faiz, Mohd Azri and Puan Farah for their help in this study.
conflict of interest
The authors declare that there is no conflict of interest regarding the publication of this paper.
authors contribution
HAH and NDR conceived of the presented idea. NDR carried out the experiment and wrote the manuscript. NDR and AAAG analysed the data. HAH, MTY and MZS supervised the project. HAH, MTY, MZS and KM reviewed and revised the manuscript.
REFERENCES