Advances in Animal and Veterinary Sciences
Research Article
Hypothalamic, Hepatic and Renal Tricarboxylates in HFHS-Induced Metabolic Syndrome in Rats: A Molecular Study
Khalifa El-Dawy1, Ashraf S. Abdel Fattah2, Sara A. Elhefnawy1, Ahmed Hamed Arisha3*
1Department of Biochemistry, Faculty of Veterinary Medicine, Zagazig University, 44519 Zagazig, Egypt; 2Department of Microbiology, Faculty of Science, Zagazig University, Zagazig, Egypt; 3Department of Physiology, Faculty of Veterinary Medicine, Zagazig University, 44519 Zagazig, Egypt.
Abstract | Both the central and peripheral regulation of energy balance involves several complex processes where most of which remain unexplored. The main objective of this study was to explore the potential molecular implication of several transporters and the enzymes involved in the tricarboxylic acid cycle (TCA), electron transport chain (ETC) and de novo glycine biosynthesis in the hypothalamus, liver and kidney along with, in particularly, the expression analysis of the mitochondrial citrate carrier 1 and 2 (CIC1 and CIC2), isocitrate dehydrogenase 1 and 2 (IDH 1 and IDH 2), cytochrome C oxidase (Cyt-C or complex IV) and serine hydroxymethyl transferase (GlyA or SHMT) in high fat high sugar (HFHS)-induced metabolic syndrome (MetS) in male Sprague dawely rats. Moreover, the metabolic effects of the oral administration of gum arabic (GA) and/or the hypoglycemic drug (dapagliflozin) were studied. Fifty rats were randomly divided into 5 groups; control group, HFHS-induced MetS group and HFHS-induced MetS-supplemented with either gum arabic (500mg/kg) and/or administered with dapagliflozin (1mg/kg). Furthermore, studying the gene expression pattern of the hypothalamic AMP-activated protein kinase (AMPK), acetyl-coA carboxylase (ACC) and fatty acid synthesis (FAS) involved in the central regulation of energy 16 balance, in such experimental models. Our results showed significant up-regulation in the hypothalamic mRNA expression of AMPK while ACC and FAS expression were down-regulated in the HFHS- induced MS compared to the control group. Furthermore, a marked difference was observed in the rate of expression of the CIC1, CIC2, IDH1, IDH2, Cyt-C and GlyA expression level in the hypothalamic, hepatic and renal tissues of HFHS- induced MetS. Oral supplementation of GA and/or treatment with dapagliflozin reverted most of these changes while the combination group had the most evident effect. In conclusion, molecular targets such as studied herein could provide a valid therapeutic potential and explain the mechanisms of the energy imbalance accompanied by MetS/ diabetes dilemma.
Keywords | CIC, IDH, GlyA, Cyt-C, Metabolic syndrome (MetS)
Received | September 19, 2019; Accepted | October 26, 2019; Published | December 12, 2019
*Correspondence | Ahmed Hamed Arisha P.h.D., Department of Physiology, Faculty of Veterinary Medicine, Zagazig University, 44519 Zagazig, Egypt; Email: [email protected]
Citation | El-Dawy K, Fattah ASA, Elhefnawy SA, Arisha AH (2019). Hypothalamic, hepatic and renal tricarboxylates in hfhs-induced metabolic syndrome in rats: A molecular study. Adv. Anim. Vet. Sci. 7(s2): 86-95.
DOI | http://dx.doi.org/10.17582/journal.aavs/2019/7.s2.86.95
ISSN (Online) | 2307-8316; ISSN (Print) | 2309-3331
Copyright © 2019 El-Dawy et al. This is an open access article distributed under the Creative Commons Attribution License, which permits unrestricted use, distribution, and reproduction in any medium, provided the original work is properly cited.
INTRODUCTION
The regulation of energy balance involves a complex network of interactions between numerous factors such as the circulating nutrients, hormones, peptides, and neuropeptides. Energy homeostasis assures a stable balance between energy consumed and expended. This balance is established via metabolic communications between several central and peripheral regulators (Lopez et al., 2007; Wren and Bloom, 2007). Among these, the hypothalamic, hepatic and renal cellular metabolism, metabolic signaling and effect on the overall body metabolism are prominent contributors to the net metabolic state. Noticeably, obesity has developed into an issue of considerable concern. Generally, overweight gives rise to the development of metabolic syndrome (MetS or syndrome X). Although the pathophysiology of the MetS is not completely understood, it has been found to be linked to an elevated incidence of cardiovascular diseases, insulin resistance, diabetes mellitus and neurological complications (Catharina et al., 2018).
Recently, some basic cellular metabolic pathways demonstrated a remarkable part in this physiological network. AMP‐activated protein kinase (AMPK) is the core energy sensor in any given cell. Activation of AMPK following cellular energy stores depletion is essential for proper energy homoeostasis. At a hypothalamic level, the AMPK pathway, including the acetyl‐CoA carboxylase (ACC) and fatty acid synthase (FAS), is believed to integrate the peripheral hormonal and several metabolic signals (López, 2018; Lopez et al., 2007). Hypothalamic AMPK is directly linked to changes in feeding behavior, brown adipose tissue (BAT) activity, browning of white adipose tissue (WAT), liver metabolic function and muscle metabolisms (López, 2018). Given their vital function in cellular metabolism, mitochondria are crucial for the pathophysiology of diabetes. The full implication of aerobic cellular respiration pathways including glycolysis, TCA) and oxidative phosphorylation containing the electron transport chain (ETC) and chemiosmosis (Anderson et al., 2018) as well as the transporters involved in such mechanisms remains understudied; both at central and peripheral levels. The TCA, a prime metabolic pathway for different aerobic processes in an animal tissue and ultimate pathway for the oxidation of carbohydrates, lipids and proteins, is essential for gluconeogenesis, transamination, deamination and lipogenesis (Melendez-Hevia et al., 1996).
Nowadays different treatments such as insulin therapy, pharmacotherapy, and diet therapy are followed to control diabetes. Numerous glucose-lowering medications exert anti-diabetic effects via several mechanisms (Bathaie et al., 2012; Hui et al., 2005). These therapeutics potentially give rise to various secondary complications including cardiovascular diseases, kidney failure, liver injury and mental disorders (Mohammed et al., 2013). Dapagliflozin, the sodium glucose co-transporter-2 (SGLT-2) inhibitor, reduces blood glucose level in diabetic individual regardless of insulin level. It acts via suppressing renal glucose reabsorption and increasing urinary glucose excretion (Jabbour et al., 2018). Therefore, an appropriate management of T2DM should include a combination between pharmacotherapy and healthier lifestyle choice of a balanced dieting and exercise (Franz et al., 2010). Furthermore, natural products and its derived bioactive molecules may possibly represent proper alternatives for the drug treatment of T2DM without adverse effects. Tremendous amount of medicinal plants and its natural active principles have been reported to possess a certain degree of therapeutic nature against diabetes (Patel et al., 2012). Several medicinal plants have been used in folk medicine to control diabetes and associated conditions (Arulselvan et al., 2014).
Gum Arabic (Acacia Senegal, GA) is a water-soluble dietary fiber with minimal digestibility in humans or animals. GA contains a mixture of polysaccharides, oligosaccharides and glycoproteins. It has the ability to delay the absorption and digestion of carbohydrates thus contributing a body weight reduction and glycemic control (Ahmed, 2018; Babiker et al., 2017; Babiker et al., 2018). The main objective of this study was to explore the potential molecular implication of the mitochondrial citrate carrier 1 and 2 (CIC1 and CIC2), isocitrate dehydrogenase 1 and 2 (IDH1 and IDH2), cytochrome c oxidase (Cyt-C or complex IV) and serine hydroxymethyl transferase (GlyA or SHMT) in the hypothalamic, hepatic and renal tissues of HFHS-induced MetS in male Sprague Dawley rats orally administered with gum arabic (GA) and/or a hypoglycemic drug (dapagliflozin).
Materials and Methods
Experimental Animals
Fifty male Sprague Dawley rats (150±30 gm) were obtained from the central animal house of Faculty of Veterinary Medicine, Zagazig University. All animal experiments were conducted following the procedures approved by the Zagazig University Institutional Animal Care and Use Committee (IACUC) NO. (ZU-IACUC/2/F/94/2019).
Drugs and diet preparations
Gum Arabic powder was obtained from a trusted local market, purified and sieved from any impurities. The fine powder was dissolved in distilled water, prepared daily and the solution was given to rats at a dose of 500 mg/kg of body weight per day during the 4 weeks of the experiment (Georgiadis et al., 2014). Dapagliflozin tablets were crushed, dissolved in distilled water and orally administered to the rats at a dose of 1 mg/kg for 4 weeks (Han et al., 2008). Each 1 kg of diet composed of lard (350 g), fructose (170 g) and 480g chow.
HFHS- induced metabolic syndrome rat model
Induction of metabolic syndrome was made according to previously described protocol (King and Austin, 2017; Nakajima et al., 2015; Wong et al., 2016) by feeding the prepared diet and offering drinking water containing 10% sucrose. The rats were housed for 8 weeks. Body weight and fasting blood glucose were determined once per week. This animal model has been previously validated and reported by our study group (Alaa, 2019; El-naggar and El-Dawy, 2019; Elkahky, 2019).
Experimental design
Fifty rats were randomly divided into 5 groups with each of 10 rats as follow: a. control group: fed on standard rat diet and water for 12 weeks, b. HFHS-induced MS group: fed on HFHS diet for the 12 weeks period of experiment, c. HFHS- induced MetS-supplemented with gum arabic (500mg/kg) during the last 4 weeks, d. HFHS- induced MetS-administered with dapagliflozin (1mg/kg) during the last 4 weeks and e. HFHS- induced MetS-co-administered with gum arabic (500mg/kg) and dapagliflozin (1 mg/kg) during the last 4 weeks.
Sampling
At the end of experimental period, blood samples were collected from each rat via median eye can thus in sodium fluoride for measuring blood glucose level and without anticoagulant for serum hormonal assays. Serum glucose was determined by oxidase method using Spectrum Diagnostics glucose kit (Spectrum Diagnostics, Egypt) (El-Gayar et al., 2012) and serum insulin level was estimated using ELISA rat insulin kits method (Ray Biotech) (Wang et al., 2013) following manufactures instructions. Immediately after sacrificial procedure, the hypothalamus, kidney and liver were excised, wrapped in aluminum foil and immediately snap-frozen in liquid nitrogen for RT-PCR analysis for the gene expression.
RT-PCR
The used real time PCR assay has been previously reported (Arisha et al., 2019; Khamis et al., 2020). Briefly, total RNA was extracted from the hypothalamus, liver and kidney using Trizol (Invitrogen; Thermo Fisher Scientific, Inc.) (Arisha and Moustafa, 2019) and the cDNA synthesis was performed using the Hi Sen Script™ RH (-) cDNA Synthesis Kit (iNtRON Biotechnology Co., South Korea) in a Veriti 96-well thermal cycler (Applied Biosystems, Foster City, CA). For analysis of gene expression, using the specific primers (Table 1), the real-time RT-PCR was performed in a Mx3005P Real-Time PCR System (Agilent Stratagene, USA) using 5x HOT FIRE Pol Eva Green qPCR Mix Plus (Solis Bio Dyne, Tartu, Estonia) with initial denaturation at 95 °C for 12 minutes followed by 40 cycles of denaturation at 95 °C for 15 seconds, annealing at 60 °C for 30 seconds, and extension at 72 °C for 30 seconds. The expression level of the target genes listed in Table 1 was normalized using the mRNA expression of two known house-keeping genes (β-actin and GAPDH) as recommended by the MIQUE guidelines (Bustin et al., 2009). The ΔΔCT Cp-method with the geometric mean of the reference genes for normalization was performed (Hellemans et al., 2007). Results are expressed as fold-changes compared to the control group (Livak and Schmittgen, 2001).
Statistical analysis
One-way analysis of variance (ANOVA), followed by Post hoc Tukey test was used. Analysis was done using GraphPad prism 7 (GraphPad Software Inc., San Diego, 189 CA, USA). Results were reported in means ± SEM (Standard Error of Mean). The value of P < 0.05 was used to indicate statistical significance.
Table 1: Primers Sequences used for real time PCR.
Gene | Primers | |
CIC1 | F | AGTCTTCACGTATTCGGTCTC |
R | CCGAATACGTGAAGACTCATT | |
CIC2 | F | CCGTGAAGGTGAAATTCATTT |
R | GCTACTGTACTGAAGCAGGTT | |
IDH1 | F | CTCTGTGGCCCAAGGGTATG |
R | GGATTGGTGGACGTCTCCTG | |
IDH2 | F | CCTGCTGTTCGCTCTCC |
R | GCTTCGCCACCTTGATCCT | |
Cyt-C | F | TCGCCGACCGTTGACTATTCTCT |
R | AAGATTATTATAAATGCATGGGC | |
GlyA | F | CAAAAGCAGTTATGGCAGCA |
R | ACATCAATTGCTTCTGTTCTC | |
AMPK | F | CTCGCCCAATTATGCTGCAC |
R | GGGAGAGTTCCACACAGCAA | |
ACC | F | AACAGTGTACAGCATCGCCA |
R | CATGCCGTAGTGGTTGAGGT | |
FAS | F | GAGTATACAGCCACCGACCG |
R | AGTTGCACACCACAAGGTCA | |
GAPDH | F | TCCCTCAAGATTGTCAGCAA |
R | AGATCCACAACGGATACATT | |
β-actin |
F | CCACCATGTACCCAGGCATT |
R | CGGACTCATCGTACTCCTGC |
RESULTS
Hypothalamic mRNA expression:
A significant upregulation (P < 0.01) in the hypothalamic expression of AMPK and down-regulation (P<0.01) in the expression of ACC and FAS were noticed in the HFHS induced MetS group compared to control rats (Figure 1). Supplementation of diabetic rats with GA powder (500mg/kg) showed a significant down-regulation (P<0.05) in AMPK and up-regulation (P<0.01) of ACC (Figure 1). Treatment of diabetic rats with dapagliflozin (1mg/kg) significantly down-regulated (P<0.001) the hypothalamic expression of AMPK and up-regulated (P < 0.01) ACC and FAS compared to the diet induced MS group (Figure 1). A more ameliorative effect was obtained by the combination between dapagliflozin with GA (Figure 1).
The hypothalamic gene expression of CIC1, IDH1, and Cyt-C were down-regulated (P<0.001), whereas no significant alteration in the expression of CIC2, IDH2 and GlyA were noticed in the HFHS- induced MetS group (Figure 1). Supplementation of diabetic rats with GA powder (500mg/kg) and/or treatment with dapagliflozin (1mg/kg) significantly up-regulated (P<0.01) the hypothalamic expression of CIC1, IDH1, and Cyt-C compared to the MetS group (Figure 1). The combination between dapagliflozin with GA significantly up-regulated (P < 0.001) the hypothalamic expression of CIC1, CIC2, IDH1, IDH2 and Cyt-C but not GlyA compared to the MetS group (Figure 1).
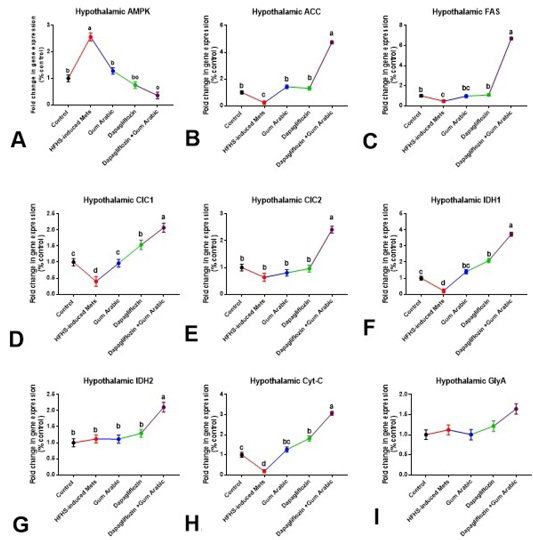
Figure 1: Hypothalamic changes in gene expression (A-I). A. Hypothalamic AMPK, B. Hypothalamic ACC, C. Hypothalamic FAS, D. Hypothalamic CIC1, E. Hypothalamic CIC2, F. Hypothalamic IDH1, G. Hypothalamic IDH2, H. Hypothalamic Cytc and I. Hypothalamic GlyA. Relative expression was calculated as a percentage of the control value. Data are presented as means ± SEM. Means bearing different superscripts were significantly different at P < 0.05.
Hepatic mRNA expression
The hepatic gene expression of CIC1, IDH1, IDH2, Cyt-C and GlyA were significantly down-regulated (P < 0.01) in the HFHS induced MetS group compared to control rats (Figure 2). Supplementation of diabetic rats with GA powder significantly up-regulated (P<0.05) the hepatic expression of CIC1, IDH1, IDH2 and GlyA compared to the MetS (Figure 2). Treatment of diabetic rats with dapagliflozin (1mg/kg) as well as the combination between dapagliflozin with GA induced a more effective ameliorative effect as shown in Figure 2.
Renal mRNA expression
The renal gene expression of CIC1, CIC2, Cyt-C and GlyA was significantly down-regulated (P<0.01) in HFHS-induced MetS compared to control (Figure 3). Supplementation of diabetic rats with GA powder (500mg/kg) significantly up-regulated (P<0.001) the renal expression of CIC1, Cyt-C and GlyA compared to MetS (Figure 3). Also, treatment of diabetic rats with dapagliflozin (1mg/kg) significantly up-regulated (P < 0.001) the renal expression of CIC1, CIC2, IDH1 Cyt-C and GlyA compared to the MetS group (Figure 3). The combination between dapagliflozin with GA induced a more effective ameliorative effect as shown in Figure 3.
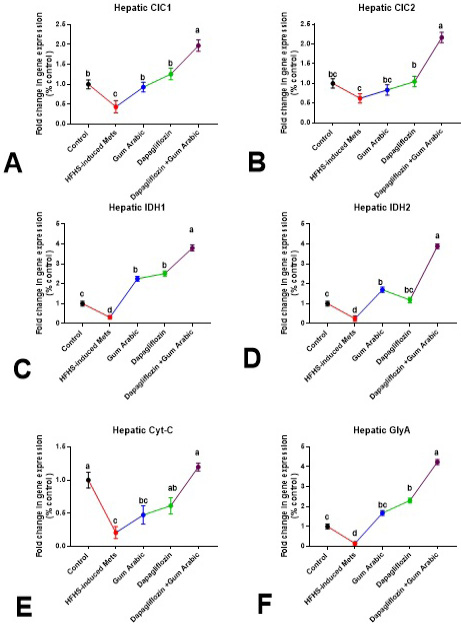
Figure 2: Hepatic changes in gene expression (A-F). A. Hepatic CIC1, B. Hepatic CIC2, C. Hepatic IDH1, D. Hepatic IDH2, E. Hepatic Cytc and F. Hepatic GlyA.Relative expression was calculated as a percentage of the control value. Data are presented as means ± SEM. Means bearing different superscripts were significantly different at P < 0.05.
DISCUSSION
Obesity is a result of a disturbance affecting the metabolic homeostasis where excessive food consumption is accompanied by an inadequate activity. It has been linked to metabolic changes in the hypothalamus, liver and kidney. It is concomitant to co-morbidities involving cardiovascular diseases, insulin resistance, type 2 diabetes mellitus (T2DM), and even cancer (van der Klaauw and Farooqi, 2015). A common consequence of obesity is metabolic syndrome (MetS), a condition that predispose individuals to the development of cardiovascular disease and T2DM (Ye, 2013). (Choudhury et al., 2017) It constitutes a leading public health problem of the modern era. Recently, herbal plants and their extracts have been widely investigated as an alternative or supportive agent over drugs in the treatment against different diseases including T2DM (Choudhury et al., 2017). These herbal supplements have the advantage of being generally non-toxic. A standard supplementation with plant derivatives to induce hypoglycemia has been practiced in folk medicine from very ancient time. Nonetheless, the beneficial effects of medicinal plants on pancreatic dysfunction in diabetic patients have been widely reported (Assaei et al., 2016). The present study provides novel insights on the central and peripheral mechanisms involved in the development of HFHS-induced metabolic syndrome as well as investigating the effect of gum arabic alone or in combination with a hypoglycemic therapeutic agent (dapagliflozin) to treat diet induced MetS.
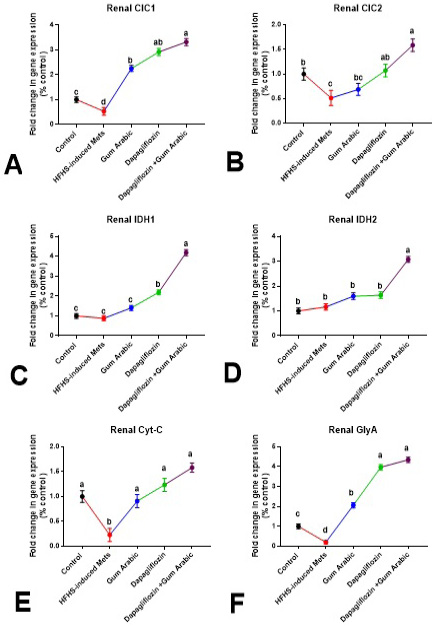
Figure 3: Renal changes in gene expression (A-F). A. Renal CIC1, B. Renal CIC2, C. Renal IDH1, D. Renal IDH2, E. Renal Cytc and F. Renal GlyA. Relative expression was calculated as a percentage of the control value. Data are presented as means±SEM. Means bearing different superscripts were significantly different at P<0.05.
Animal models are essential for proper understanding of the pathophysiology of type 2 diabetes mellitus (T2DM), MetS and its associated complications (Arndt et al., 2013). Manipulation of the dietary composition by feeding a high energy diet is a valid approach for inducing obesity and its associated complications in rodents. This model reflects the mechanism of obesity-induced diabetes in humans (Gilbert et al., 2011; King and Bowe, 2016). This animal model has been extensively studied and validated by our group as described in (Alaa, 2019; El-naggar and El-Dawy, 2019; Elkahky, 2019). The hypothalamic inflammation has been reported as a vital step in the development of such complications, the exact molecular mechanisms underlying this response of hypothalamic neurons to HFD remains not fully understood. Remarkably, the hypothalamic inflammatory response was clear within days of the consumption of a HFD long before any significant weight change in rodents (Thaler and Schwartz, 2010).
Hypothalamic AMPK and its associated pathway have emerged as a key regulator in energy metabolism and lipid homeostasis (Lopez, 2017). Both fasting and high-fat dieting (HFD) were reported to increase the activity of AMPK in the hypothalamus (Cavaliere et al., 2018; Martin et al., 2006; McCrimmon et al., 2004). In contrast, feeding and hypothermia were reported to decrease AMPK activity in the hypothalamus (Cao et al., 2017; McCrimmon et al., 2004). Consequently, the change in AMPK activity modulates the activity of acetyl CoA carboxylase (ACC) (Choi et al., 2017; Li et al., 2014) as well as fatty acid synthase (FAS) (López, 2018; Lopez et al., 2007). However, six weeks dietary consumption of HFD did not modify the hypothalamic expression of AMPK although an increase in the level of pAMPK was noticed in rats (Viggiano et al., 2016). Furthermore, this study has reported for the first time a potential protective effect of the dietary supplementation of GA powder at a dose of 500mg/kg/day alone or in combination 1mg/kg/day dapagliflozin for MetS rats. In this experiment, a significant up-regulation have been reported in the hypothalamic expression of AMPK, whereas down-regulation in the expression of ACC and FAS was observed in the HFHS-induced metabolic syndrome group compared to control rats. Also, supplementation of diabetic rats with GA powder significantly down-regulated AMPK and up-regulated ACC expression compared to the diet induced Mets group. Treatment of diabetic rats with dapagliflozin significantly down-regulated the hypothalamic expression of AMPK and up-regulated ACC and FAS compared to the diet induced Mets group. The combination between dapagliflozin with GA induced a more ameliorative effect.
The mitochondrial carriers (MCs), the membrane embedded proteins, are found in the inner membranes of mitochondria and catalyze a proper selective transport process of certain metabolites to allow appropriate link between cytosol and mitochondria. This link is crucial for all the physiological processes involving the activities of both intra‐ and extra-mitochondrial enzyme (Palmieri, 2004). In animals, the citrate (or tricarboxylate) carrier (CIC) members essential to catalyze the electro-neutral passage of a tricarboxylate in exchange for another tricarboxylate across the inner mitochondrial membrane are well‐characterized (Bisaccia et al., 1989; Bisaccia et al., 1990; Zara et al., 1996). These carrier proteins export citrate from the mitochondria to the cytosol (Dolce et al., 2011). In general, the different CIC members are involved in fatty acid synthesis, gluconeogenesis, insulin secretion, and inflammation (Cappello et al., 2012; Joseph et al., 2006; Siculella et al., 2004). Although limited literature discusses the potential of CIC regulation in coordination with the metabolic pathways to which it either provides substrate or eliminates product. In diabetic rats, CIC activity has been reported to be influenced by hormonal status as well as nutritional factors (Capobianco et al., 1995; Iacobazzi et al., 1996; Persson, 2000). A decreased CIC transport activity was reported in type 1 diabetic rat liver compared with the non-diabetics (Capobianco et al., 1995). This decreased activity was restored via insulin administration (Persson, 2000). The results of this study demonstrated a significant down-regulation in the hypothalamic, hepatic and renal expression of CIC1 as well as the renal but not hypothalamic or hepatic expression of CIC2 in the HFHS induced MetS group. Overall, the combination between dapagliflozin with GA induced a more effective ameliorative effect regarding CIC expression.
Isocitrate dehydrogenases (IDHs) play vital roles in cellular metabolism. It catalyzes the oxidative decarboxylation of isocitrate in the TCA cycle (Gabriel et al., 1986). In mammalian tissues, although both IDH1 and IDH2 are moderately expressed, IDH1 is highly expressed in the liver andIDH2 is highly expressed in heart and muscle tissues (Jennings et al., 1994). IDH1 is localized in the cytoplasm (Geisbrecht and Gould, 1999; Yoshihara et al., 2001) and IDH2 is mitochondrial (Nekrutenko et al., 1998). Both IDH1 and IDH2 contribute to the defense against oxidative stress. However, the activity of both enzymes is reduced with the accumulation of lipid peroxidation products (Kim et al., 2004) as well as elevated ROS (Lee et al., 2001) and nitric oxide (Yang et al., 2002). This process is likely mediated in diabetic human and rat tissue by non-enzymatic glycation due to hyperglycemia (Kil et al., 2004). Overexpression of IDH in transgenic mice was associated with hyperlipidemia, fatty liver and obesity (Koh et al., 2004). The results of this study reported a significant down-regulation in the hypothalamic, hepatic but not renal expression of IDH1, although only hepatic IDH2 was significantly down-regulated in the HFHS induced MetS group. Interestingly, the combination between dapagliflozin with GA significantly upregulating both IDH1 and IDH2 at all levels.
Cytochrome C oxidase (Cyt-C) or complex IV, a component of the respiratory chain, is located in the inner mitochondrial membrane. It catalyzes the transfer of electrons from cytochrome-C to molecular oxygen. The activity of this complex is crucial for aerobic energy production; any defect in its activity induces the accumulation of lactic acid. It is highly expressed in the brain, muscle and heart (Lee et al., 1996; Salo et al., 1992; Tulinius et al., 1991). In diabetic rats, the activities of the complex III and complex IV of the ETC were significantly decreased while that of complex I and complex II were increased (Elkahky, 2019; Raza et al., 2011). This observation was further confirmed in our present study. Also, supplementation of diabetic rats with GA powder significantly up-regulating the hypothalamic and renal but not hepatic Cyt-C expression compared to the diet induced Mets group. Treatment of diabetic rats with dapagliflozin as well as the combination between dapagliflozin with GA significantly up-regulating the central and peripheral Cyt-C expression compared to the diet induced MetS group.
Serine hydroxymethyl transferase (GlyA or SHMT) catalyzes glycine synthesis from serine. Serine is essential for the generation of the antioxidant glutathione (GSH) (Nguyen et al., 2013). Although GlyA is ubiquitously expressed, most of the GlyA-dependent glycine synthesis occurs within the liver. although, changes in the serine to glycine conversion rate have not been reported yet in obesity, metabolic syndrome and diabetes, a reduction in the plasma serine level has been reported in these metabolic disorders (Mardinoglu et al., 2014; Mardinoglu et al., 2017). This change remains controversial (Gaggini et al., 2018). In this study, no significant alterations in the hypothalamic expression of GlyA were noticed in any of the experimental groups. A significant down-regulation in both the hepatic and renal expression of GlyA in the HFHS induced MetS group. This effect was reversed via administration of dapagliflozin and/or supplementation with GA compared to the diet induced Mets group.
Conclusions and Recommendation
The ameliorative effect of dietary supplementation with gum arabic and/or treatment with dapagliflozin extended to the hypothalamic, hepatic and renal gene expression of several genes suggesting potential targets that could possibly lay basis for future therapeutics and therapeutic applications. These molecular targets could provide a valid therapeutic potential for the metabolic syndrome/diabetes dilemma.
Acknowledgments
The present study was a result of collaboration between the Departments of Biochemistry and Physiology, Faculty of Veterinary Medicine, and the Medical Research Center at the Zagazig University, Egypt.
Authors Contribution
K.E, S.A.E and A.H.A conceived the project, researched data, analyzed data and drafted the manuscript. K.E and A.S.A researched the data and reviewed and edited the manuscript. All authors read and approved the final draft of the manuscript.
Conflict of interest
The authors declare no conflicts of interest, financial or otherwise.
References