The Journal of Advances in Parasitology
Review Article
Pre-erythrocytic Stage of Malaria Infection and the Molecular Targets Available for Interventions
Dickson Adah1,2, Yi Jun Yang1,2, Quan Liu1,2, Limei Qin1, Li Qin1, Xiaoping Chen1*
1State Key Laboratory of Respiratory Disease, Center for Infection and Immunity, Guangzhou Institutes of Biomedicine and Heath, Chinese Academy of Sciences, 190 Kaiyuan Avenue, Science Park, Guangzhou 510530, PR China; 2University of Chinese Academy of Sciences, No. 190 Yuquan Road, Beijing 100049, PR China.
Abstract | Despite tremendous efforts aimed at eradicating malaria, the disease is still a global health challenge. The ability of Plasmodium species to develop resistance, evade and escape host immunity makes treatment and vaccine development a difficult task. The pre-erythrocytic stage of malaria infection presents ample opportunity for effective sporozoites clearance due to low sporozoites number and comparative clinical silence. Among the current malaria vaccines in clinical trials, RTS,S/AS01 is the most advanced malaria vaccine candidate that has shown promise, and it is known to target the pre-erythrocytic stage. This highlights the importance of targeting early infection. In recent years, there has been tremendous progress in this field, and several host and parasite derived proteins show strong potential as malaria vaccine targets. Current knowledge in this field is reviewed with the aim of highlighting opportunities available for effective malaria interventions.
Keywords | Malaria, Sporozoites, Invasion, Molecular targets, Vaccines
Editor | Muhammad Imran Rashid, Department of Parasitology, University of Veterinary and Animal Sciences, Lahore, Pakistan.
Received | July 04, 2016; Accepted | August 26, 2016; Published | September 06, 2016
*Correspondence | Xiaoping Chen, State Key Laboratory of Respiratory Disease, Center for Infection and Immunity Guangzhou Institutes of Biomedicine and Health, Chinese Academy of Sciences, 190 Kaiyuan Avenue, Science Park, Guangzhou, 510530, PR China; Email: chen_xiaoping@gibh.ac.cn
Citation | Adah D, Yang YJ, Liu Q, Qin L, Qin L, Chen X (2016). Pre-erythrocytic stage of malaria infection and the molecular targets available for interventions. J. Adv. Parasitol. 3(4): 132-141.
DOI | http://dx.doi.org/10.14737/journal.jap/2016/3.4.132.141
ISSN | 2311-4096
Copyright © 2016 Adah et al. This is an open access article distributed under the Creative Commons Attribution License, which permits unrestricted use, distribution, and reproduction in any medium, provided the original work is properly cited.
Malaria remains the most important parasitic disease in humans, affecting nearly half of the human population. Among the several Plasmodium species that cause human malaria, Plasmodium falciparum (P. falciparum) accounts for the majority of severe malaria and deaths, followed by Plasmodium vivax(P. vivax) (Beeson et al., 2016). Malaria parasites have both asexual (which takes place in the mammalian host), and sexual (which takes place in the anopheles mosquitoes) phases in life cycle. The erythrocytic cycle generates male and female gametocytes which are picked up by mosquitoes during a blood meal, leading to fertilization and zygote formation within the mosquito stomach (Zhang et al., 2010). Motile zygote (ookinete) penetrates the midgut epithelium to form oocysts within which hundreds of sporozoites are generated. Oocysts rupture to release sporozoites which invade the salivary glands from where they are injected into the mammalian blood during a bite. Within minutes of sporozoites inoculation in mammalian host, rapid and efficient hepatocytes invasion are believed to take place (Shin et al., 1982). While in the liver cells, sporozoites multiply and differentiate into merozoites which are subsequently released to bind and invade erythrocytes (Sultan, 1999). The differentiation of a certain population of merozoites into gametocytes and subsequent uptake of male and female gametocytes by mosquitoes ensures the passage from asexual to sexual stage of Plasmodium development.
Malaria intervention strategies involving the use of insecticide-treated-bed nets, improved access to early diagnosis and the use of highly effective Artemisinin -based combination therapy (ACT) has yielded tremendous results in reducing the burden of malaria globally (Beeson et al., 2016). However, despite the availability of preventive and curative drugs, eradication still remains a mirage due to parasite resistance (White et al., 2014). Although malaria vaccines have made some progress in recent times with some undergoing phase III clinical trials, none has gained approval to this date. To this end, identification of new targets for drug and vaccine intervention remains a top priority (Moorthy et al., 2004; Moorthy and Kieny, 2010).
The injection of sporozoites into the mammalian host through the bite of an anopheline mosquito is the first step of malaria transmission (Amino et al., 2006; Vanderberg and Frevert, 2004). The pre-erythrocytic stage of malaria infection is considered as the best target for intervention strategies which aim to prevent malaria by taking advantage of the low sporozoites number as well as their clinical silence within the human host (Aly et al., 2009; Kappe et al., 2010). Precise and effective intervention at this stage can completely abrogate Plasmodium infection. However, taking advantage of this stage of malaria infection for effective intervention requires adequate knowledge of sporozoites journey from the point of inoculation to the liver and the molecular mechanisms of liver infectivity.
Over the past few years, several host and parasite derived proteins has been identified and known to play a role in sporozoites migration, invasion and development in the liver. In this review, we summarized the recent understanding of the roles of these proteins as well as the molecular mechanisms involved in hepatocytes infectivity. We also highlighted existing challenges facing malaria intervention strategies and current opportunities available for effective interventions.
Sporozoites Migration from the Point of Inoculation to the Liver: A Key Step towards Productive infectivity
For the sporozoites to invade liver cells it has to travel from the point of inoculation to the liver and doing that will require traversing different cell types. Ability to traverse cells, move into the blood stream and avoid immune surveillance is vital to an effective migration to the liver. It was earlier believed that sporozoites are injected directly into the blood stream during mosquito bites and invade the liver cells minutes after inoculation. However, recent studies reveals that mosquitoes only injects sporozoites in saliva when they probe for blood and stops salivation when they start sucking blood from their host (Sinnis and Coppi, 2007). It is now known that majority of sporozoites from the mosquito’s salivary glands are injected into the dermis (Figure 1) and not directly into the blood stream (Matsuoka et al., 2002; Medica and Sinnis, 2005; Sidjanski and Vanderberg, 1997). While in the dermis, sporozoites have been shown to move randomly until they are able to locate and penetrate blood vessels to gain access into the blood stream (Amino et al., 2006). Blood circulates through the entire body but sporozoites are known to bind specifically to the liver cells. To understand the reason behind this affinity for hepatocytes, we need to examine what differentiates the liver cells from other cells in the body. Studies reveal that the liver has distinct blood vessels and sinusoids with walls made up of fenestrated endothelial cells and macrophage-like kupffer cells (Widmann et al., 1972). The highly vascularized nature of the liver ensures that sporozoites-infected blood is transported into the liver but other factors are responsible for their arrest, invasion and further developments.
Sporozoites Arrest, Invasion and Development In The Liver
Stellate- cell-derived extracellular cell matrix (ECM) proteoglycans protruding from the space of Disse through the endothelial sieve plates into the sinusoidal lumen are believed to mediate the initial arrest of sporozoites in the liver (Pradel and Frevert, 2001; Pradel et al., 2002).
Strong interaction between circumsporozoite protein (CSP) and the highly sulfated heparan sulphate proteoglycans (HSPG) synthesized by stellate cells in the space of Disse is believed to be responsible for sporozoites arrest in the liver (Cerami et al., 1994; Coppi et al., 2007; Frevert et al., 1993; Pradel et al., 2002). HSPG is widely distributed in many tissues but heparan sulfate in the liver exhibit unusually high level of sulfation (Lyon et al., 1994). Although sporozoites migrate through several cells in the skin and endothelium containing HSPG without invading them, they subsequently invade hepatocytes due to high levels of HSPG sulfation (Coppi et al., 2007).
Contact of the circumsporozoite protein (CSP), the major sporozoites surface protein, with cells expressing highly sulfated HSPG has been shown to activate sporozoites to begin the process of cell invasion (Coppi et al., 2007). Sporozoites are then believed to glide along the sinusoid wall until they locate a kupffer cell (Frevert et al., 2005), traverse it and subsequently infect hepatocytes. Other data in support of this hypothesis (referred to as the “gateway hypothesis”) shows that sporozoites specifically traverse kupffer cell as opposed to endothelial cells (Danforth et al., 1980; Meis et al., 1983; Pradel et al., 2002; Vreden, 1994). However, the lack of other tools for probing the events described by this hypothesis and the observation that the sporozoites in the kupffer cells sometimes have a vacuole around them raises questions concerning the certainty of this hypothesis. Moreover, studies have shown that kupffer cells are not mandatory for sporozoites crossing
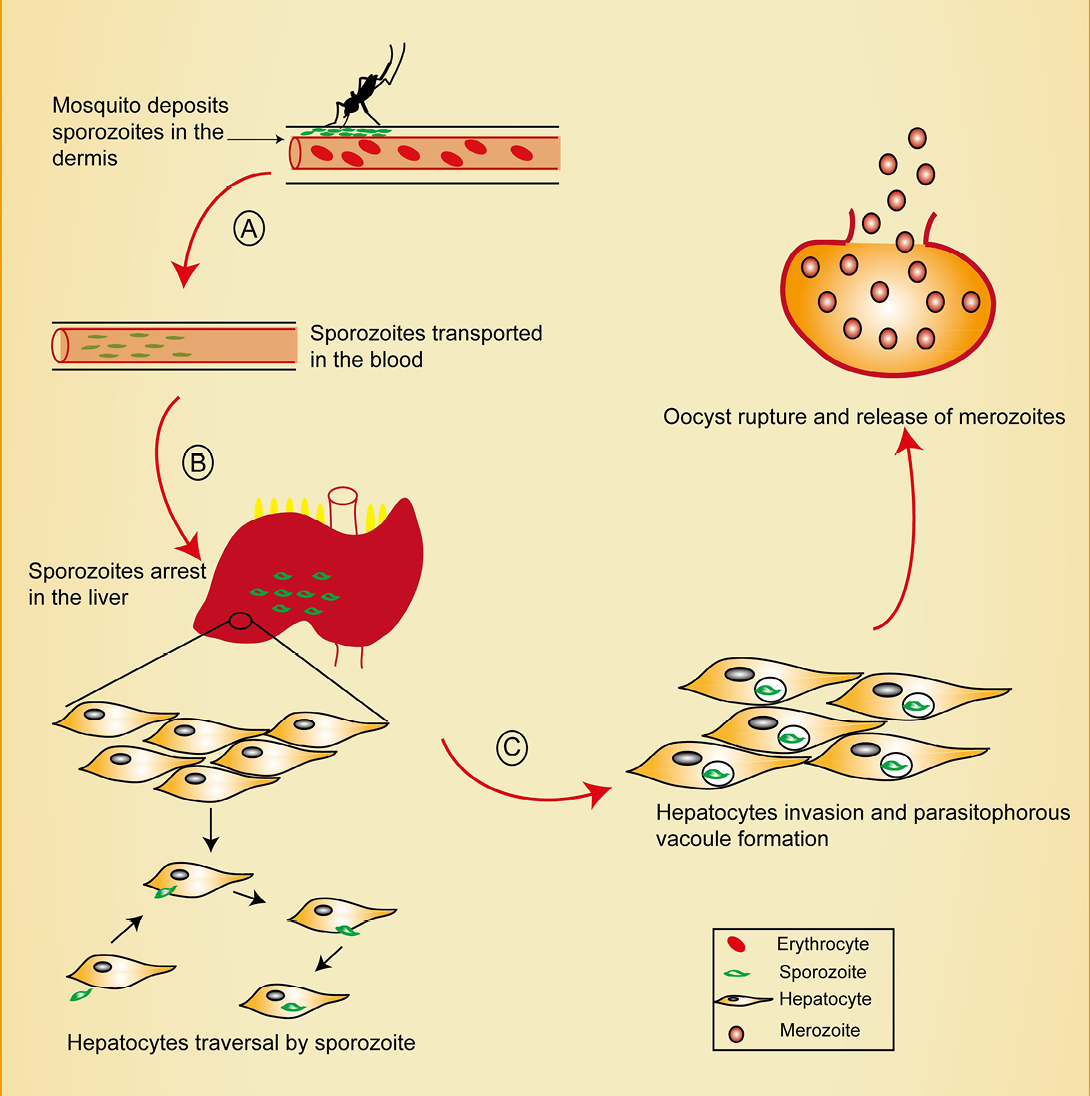
Figure 1: Pre-erythrocytic stage of malaria showing points of intervention. Mosquito deposits sporozoites in the dermis during a blood meal. Sporozoites traverse the dermis in search of a CD31+blood vessel, penetrates it and enter the blood circulation.
A) Interventions at this stage could target blocking CD31 as well as inhibition of sporozoite motility. Sporozoites migrate through kupffer cells in the liver sinusoid, a process believed to be mediated by CD68; B) Intervention at this point could target CD68 and prevent sporozoites liver entry. Sporozoites traverse several hepatocytes before finally invading one. Recent reports reveal the role of EphA2 in this selection process; C) EPhA2 targeting could inhibit productive invasion of hepatocytes.
the liver sinusoidal barrier, suggesting that Plasmodium sporozoites might cross sinusoidal barrier not only through kupffer cells but also through endothelial cells (Tavares et al., 2013).
While some authors believe sporozoites are passively engulfed by kupffer cells and then carried to the hepatocytes (Meis et al., 1983), others believe that active motility and vacuole formation are involved in the migration (Frevert, 2004; Pradel and Frevert, 2001; Pradel et al., 2004). Before finally settling down in hepatocytes for multiplication and differentiation, sporozoites are believed to be involved in migrating and breaching through several other hepatocytes, breaking their plasma membranes in the process (Mota et al., 2001). This breaching of several other hepatocytes has been shown to induce the exocytosis of sporozoites apical organelles, a process necessary for infection and vacuole formation (Mota et al., 2002). Furthermore, other researchers reports that migration through other liver cells releases hepatocytes growth factor from the wounded hepatocytes leading to increased resistance to apoptosis of surrounding hepatocytes which favors sporozoites development (Carrolo et al., 2003).
Within few hours of sporozoites hepatocytes invasion, radical transformation takes place. While in the parasitophorus vacuole (PV), the intracellular sporozoites transforms from its long and polarized shape to become spherical after settling near the nucleus of host cells (Bano et al., 2007; Jayabalasingham et al., 2010). Replication within the PV ultimately leads to the release of thousands of the membrane bound merozoites into the blood for subsequent erythrocytes invasion (Sturm et al., 2006; Tarun et al., 2006). Several proteins are known to mediate merozoites invasion of red blood cells (RBCs) but their role is poorly understood.
Challenges of previous inter-vention strategies
The ability of Plasmodium species to evade and suppress host immunity makes treatment and vaccine development a difficult task (Cohen et al., 2010). Continuous emergence and spread of resistance to newly developed antimalarials (Cohen et al., 2010; Na-Bangchang and Karbwang, 2013; Price et al., 2014; WWARN, 2015) as well as vector resistance to insecticides (Dhiman and Veer, 2014; Strode et al., 2014) are key challenges against existing malaria control measures and highlights the urgent need for an effective vaccine. Despite several preclinical and clinical trials with the aim of developing an effective malaria vaccines (Moreno and Joyner, 2015), the most advanced malaria vaccine candidate in phase 3 trial, RTS,S/AS01, has only shown 31% and 50% efficacy in infants and children respectively (Foquet et al., 2014). RTS,S/AS01 was designed based on the discovery of the sporozoites major surface protein, CSP, with the aim of interfering with the ability of the parasite to infect hepatocytes (Casares et al., 2010; Cohen et al., 2010). The major limitation of RTS,S/AS01 is that it is composed of only one surface protein, CSP and may account for its limited efficacy as individuals immunized with irradiated sporozoites has been shown to be associated with sterile protection (Trieu et al., 2011). Furthermore, complexity of the Plasmodium life cycle such that different set of antigens are presented at different stages in the host makes it difficult to design an effective malaria vaccine (de Souza, 2014; Krzych et al., 2014). To this end, there is an urgent need for more detailed understanding of the host and parasite derived proteins which play key roles in the migration and invasion of the parasite at the initial stage (pre-erythrocytic stage) of the infection.
Role of major sporozoites surface proteins in migration, invasion and disease develop-ment
Several proteins are likely to be involved in the complex sporozoites journey from the mosquito midgut to the mammalian liver but few have been identified so far. CSP was the first protein to be identified and it is known to be a multifunctional protein covering the entire surface of the sporozoites. The overall structure of this protein is conserved in all rodents, primates and human Plasmodium species with a central repeat region flanked by two conserved dormain at the N and C- terminus. The role of CSP has been well studied both in human and murine strains of Plasmodium spp. It has been demonstrated to be essential for sporozoites development (Menard et al., 1997; Thathy et al., 2002). Ability of sporozoites to egress from oocysts, invade salivary glands, exit from the dermis, and invade hepatocytes has been attributed to the NH2 and COOH-terminal regions of CSP (Coppi et al., 2005, 2011; Tewari et al., 2002; Wang et al., 2005). Several studies utilizing recombinant CSP and sporozoites expressing heterologous CSP have demonstrated the involvement of this protein in sporozoites targeting to the mosquito salivary gland and the mammalian liver (Aley et al., 1986; Cerami et al., 1992; Chatterjee et al., 1995; Frevert et al., 1993; Sidjanski et al., 1997; Sinnis et al., 1994; Tewari et al., 2002, 2005), making it a viable molecular target for vaccine development. Although RTS, S/AS01 has been shown to be a promising malaria vaccine, its limited duration of protection necessitates further studies on CSP and other sporozoites surface proteins. The development of natural immunity through the production of antibodies against a portion of the N-terminal region has been associated with a reduced risk of malaria (Bongfen et al., 2009), suggesting the importance of this target in vaccine development. The absence of this N-terminal may account for the limited duration of protection by RTS, S/AS01. Furthermore, it has been observed that specific cellular immune response to CSP, Thrombospondin-related anonymous protein/sporozoites surface protein 2 (TRAP), and liver stage antigen-1 (LSA1) were lower than that stimulated by whole parasite (Epstein et al., 2011; Seder et al., 2013) highlighting the importance of utilizing multiple targets in vaccine development.
TRAP, apical membrane antigen-1 (AMA-1), and LSA-1 are some of the other sporozoites antigens currently being tested in phase I and phase II clinical trials (Hodgson et al., 2015). TRAP, localized to the parasites micronemes (Rogers et al., 1992) is known to be essential for mosquito salivary gland invasion, sporozoites motility, and hepatocytes invasion (Ghosh et al., 2009; Robson et al., 1995; Sultan et al., 1997; Wengelnik et al., 1999). There are two conserved adhesive domains on the extracellular portions of TRAP: a von Willebrand factor A-domain (‘A-domain’) (Girma et al., 1987) and a thrombospondin type I repeat (TSR) (Lawler and Hynes, 1986). Mutation at the A-dormain impairs salivary gland invasion but not in gliding motility implying that the two processes are functionally different and further buttresses the role of the A-dormain in recognition and/or attachment to salivary gland receptor molecules (Matuschewski et al., 2002; Wengelnik et al., 1999). Released TRAP anchors to the sporozoites plasma membrane and become part of the glideosome which is a unique actomyosin -based motor complex that powers motility and invasion. Other proteins are also involved in sporozoites gliding motility. Sporozoite surface protein 3 (SSP3) is a transmembrane protein unique to Plasmodium and known to play a role in gliding motility as deletion of the protein adversely affected sporozoite gliding motility in vitro (Harupa et al., 2014).
Recent discoveries in molecular targets and opportunities available for interventions
Studying the genetics of susceptible and non-susceptible population (Zimmerman et al., 2013) have led to the discovery of host factors that mediate malaria parasite erythrocytic infection (Bartholdson et al., 2013; Cowman et al., 2012; Wright and Rayner, 2014). Unfortunately, there are no known human populations resistant to pre-erythrocytic Plasmodium infection thereby necessitating the use of animal models and in vitro studies to identify host and parasite factors that mediate pre-erythrocytic infection.
Contrary to earlier belief that sporozoites are probably injected into the blood stream, more recent studies have shown that mosquitoes deposit their saliva coated sporozoites in the dermis and that sporozoites leave the injection site slowly (Yamauchi et al., 2007) giving ample time for host and parasite interaction. Interventions at this stage (skin stage) could aim at inhibiting sporozoites motility and/or the ability of sporozoites to penetrate blood vessels. Although the mechanisms used by sporozoites to rupture the plasma membrane of host cell are not well understood, in vitro and in vivo studies have revealed the involvement of phospholipase in cell traversal process. In vivo studies using mutants in the active site of this protein delayed the prepatent period of the resulting blood infection by one day and decreased the infectivity of the parasite in the liver by 90% (Ishino et al., 2004).
TRAP and CSP are also believed to play key roles in P. berghei sporozoites exit from the dermis (Coppi et al., 2011; Ejigiri et al., 2012). Sporozoites expressing mutant CSP which lacks the N-terminus have been shown to exhibit impairment in their ability to exit the dermis. Furthermore, sporozoites carrying mutations in the putative rhomboid –cleavage site of TRAP exhibit significantly reduced gliding speed in vitro (Ejigiri et al., 2012). Interestingly, recent studies has provided evidence that CSP and TRAP are glycosylated in their thrombospondin type 1 repeat (TSR) domains (Swearingen et al., 2016), further revealing protein modification that is crucial in the design of effective antibody-based vaccines. The putative type 1 transmembrane protein PY01796 (referred to as sporozoites surface protein 3, SSP3), is another protein recently shown to be vital for sporozoites gliding motility. Recent findings has demonstrated that the lack of SSP3 lead to a defect in gliding motility (Harupa et al., 2014). Put together, recent developments in the understanding of the key proteins involved in gliding motility could provide a lead towards the development of vaccines and therapies that can inhibit the migration of sporozoites from the dermis.
Ability to locate and penetrate a blood vessel is essential for the sporozoites to migrate into the blood stream. Studies show that sporozoites recognize CD31+ blood vessels (Hopp et al., 2015) in their attempt to exit the dermis, suggesting the possibility of developing antibodies targeting this receptor that may inhibit sporozoites exit from the dermis.
In order to effectively invade hepatocytes, sporozoites migrate through kupffer cells or endothelial cells (Tavares et al., 2013). Kupffer cells are resident macrophages in the liver and are capable of carrying out sporozoites clearance yet the latter migrate through the former unharmed. Studies have shown that sporozoites carry out cell traversal motility to inhibit clearance by kupffer cells during locomotion inside the sinusoid lumen before crossing the barrier (Tavares et al., 2013). This process of host cell traversal is believed to be mediated by a novel microneme protein, named sporozoites microneme protein essential for cell traversal (SPECT) and that the disruption of the SPECT gene significantly reduced sporozoites liver infectivity (Ishino et al., 2004). This micronemal component include several attachment proteins, secreted from the apical pore during parasite movement and are translocated backwards along the parasite cell surface by actomyosin motors of the parasite. Researchers believe that this surface movement generates traction for parasite invasive motility. Furthermore, other researchers have reported the involvement of a protein with a membrane attack complex/perforin (MACPF)-related dormain in the sporozoites cell traversal process. Gene disruption of this molecule reveals the importance of this protein in sporozoites membrane-wounding activity as well as its involvement in the traversal of the sinusoidal cell layer before infecting the hepatocytes (Ishino et al., 2005). Put together, these reports suggest that parasite proteins mediating host cell traversal may be ideal antibody targets against the parasite.
Complete development of sporozoites in the liver and subsequent egress from hepatocytes is a vital process in the life cycle of the parasite. The 10 Plasmodium 6-cys proteins are known to play critical roles throughout parasite development and are considered as vital molecular targets for anti-malaria interventions. Recently, two additional proteins, sequestrin and B9, have been shown to play critical roles in Plasmodium liver-stage development as P. yoelli, P. berghei and P. falciparum mutants lacking B9 expression failed to fully develop in the liver (Annoura et al., 2014). Effective parasite growth arrest in the liver will be a vital preventive strategy as it inhibits the parasite from entering the highly complicated erythrocytic stage.
The ability of merozoites to egress from hepatocytes is necessary for the initiation of the erythrocytic stage. Merozoites egress from hepatocytes is a two-step event. The first step involves the rupturing of the parasitophorus vacuole membrane (PVM) (while leaving the hepatocytes plasma membrane (HPM) intact), and inducing the formation of merosomes (Sturm et al., 2006; Thiberge et al., 2007). The HPM-bound merosomes escapes phagocytosis by liver macrophages and moves into the lung microvasculature (which is a safer environment), where it ruptures and release hepatic merozoites (Baer et al., 2007; Sturm and Heussler, 2007). Plasmodium subtilin-like SUB1 protease has been known to play a dual role of enabling merozoites egress from both the hepatocytes and the erythrocytes. Recent studies using conditional mutagenesis in P. berghei reveals the involvement of Plasmodium SUB1 protease in this hepatic stage egress as SUB1- deficient parasites failed to rupture the PVM and to egress from hepatocytes (Tawk et al., 2013). SUB1 could therefore be an attractive multistage target for prophylactic and therapeutic anti-Plasmodium intervention strategy.
Several host cell receptors have been identified to facilitate sporozoites infection of hepatocytes including the scavenger receptor B1 (SR-B1) (Yalaoui et al., 2008), the tetraspanin CD81 (Silvie et al., 2003), and the HSPGs (Coppi et al., 2007; Frevert et al., 1993), but none of these are entirely indispensable in vivo (Kaushansky, et al., 2015). More recent studies have identified a peptide, P39 that can strongly bind to CD68 on kupffer cell surface leading to inhibition of sporozoites entry (Cha et al., 2015). Further studies on this peptide could lead to the discovery of drugs or vaccines that can prevent sporozoites liver invasion. Furthermore, Plasmodium sporozoite low- complexity asparagine-rich protein, SAP1 (Sporozoite Asparagine-rich Protein 1) have been shown to play essential role in sporozoite liver infection as targeted deletion of SAP1 in P. yoelli resulted in mutants that cannot initiate liver state development in vitro and in vivo despite its ability to traverse and invade hepatocytes(Aly et al., 2008). More importantly, the researchers observed that immunizations with Pysap1(-) sporozoites confer long-lasting sterile protection against wild-type sporozoite infection suggesting that SAP1-deleted sporozoites could be a whole cell vaccine candidate.
Sporozoites are now known to traverse several hepatocytes before finally invading a suitable one for productive infection. Recently, researchers found that sporozoites productively infect hepatocytes with high EphA2 expression and that deletion of EphA2 protected mice from liver infection (Kaushansky et al., 2015). Put together, these discoveries could present novel antibody target for vaccination against the parasite.
Conclusions
Malaria is the most dreadful human parasitic disease, putting half the human population at risk. Despite the development of effective drugs against the malaria parasites, emergence and spread of resistance to newly developed drugs over time as well as vector resistance to insecticides continues to hamper efforts to eliminate the disease. Plasmodium species continue to develop ways to evade and suppress host immunity thereby making drug treatment and vaccine development a difficult task. Malaria prevention seems to be the most promising strategy towards malaria eradication. A lot of progress has been made in the area of vaccine development despite disappointing results from majority of the vaccine candidates. Notably, no blood-stage vaccine has reached a phase III trial (Miura, 2016). Among the current malaria vaccines in clinical trials, RTS,S/AS01 has shown most success and it is known to target early pre-erythrocytic stage (Hill, 2011), further stressing the importance of targeting early infection. The pre-erythrocytic stage of malaria infection presents ample opportunity for effective sporozoites clearance due to low sporozoites number and comparative clinical silence. Recent studies have provided insights into host and parasite derived proteins which mediate parasite migration, invasion, and development in the liver. Understanding the roles of these molecular targets could lead to the development of vaccines and drugs that could effectively eradicate malaria in the nearest future.
Acknowledgements
D.A is sponsored by UCAS Fellowship for international PhD students.
Conflict of interest
The authors declare there are no conflict of interests.
Authors’ contributions
All authors contributed equally.
References