Advances in Animal and Veterinary Sciences
Research Article
Microstructure Adaptation in Mammalian Corne a Comparative Study
Zeinab Abdelftah, Ahmed Ragab, Rasha E. Abo-Eleneen, Ahlam M. EL-Bakry*
Zoology Department, Faculty of Science, Beni-Suef University, Beni-Suef, Egypt.
Abstract | Due to the epithelial ion barrier, the stromal latticelike structure of collagen fibrils, and the endothelial cells, the corneas of most vertebrate’s species stay transparent. The corneal epithelium, the corneal endothelium, or both, play an important role in controlling corneal hydration, depending on the species. Three mammalian families [Pteropodidae (Rousttus egyptiacus), Leporidae (Oryctolagus caniculus; Muridae (Rattus norvigerious)] of the present study were collected from different locations of Egypt. The investigated cornea was dissected free from the orbit and prepared for light and scanning electron microscopy and special stain. Three investigated mammalian species composed of three identical layers; epithelium, stroma, and endothelium besides appearance of Bowmen’s membrane in Rousttus egyptiacus and Oryctolagus caniculus. On the other hand, descemet’s membrane appear only in Oryctolagus caniculus. The investigated epithelial layers showed moderate affinity to stain with by Periodic Acid Schiff stain in Roussttus egyptiacus and Oryctolagus caniculus, although high activity with PAS stain in Rattus nirvigurios. Their corneal epithelium cells distinguished by irregular polygonal cells covered with moderated density of shorted microplicae. The ultimate goal is to keep the cornea transparent and provide the greatest refract. According to the rabbit cornea and its adaptive trends have eloquently described the vertebrate eye’s relative optical features. The study presents a brief image/account of certain structures of cornea for three of mammalian’s species and to correlate them with the different circumstances and variation of habitats.
Keywords | Cornea, Mammals, SEM, Stroma, Microprojections
Received | August 03, 2021; Accepted | September 29, 2021; Published | November 15, 2021
*Correspondence | Ahlam M. EL-Bakry, Zoology Department, Faculty of Science, Beni-Suef University, Beni-Suef, Egypt; Email: amalbakry2@yahoo.com
Citation | Abdelftah Z, Ragab A, Abo-Eleneen RE, El-Bakry AM (2021). Microstructure adaptation in mammalian cornea a comparative study. Adv. Anim. Vet. Sci. 9(12): 2234-2246.
DOI | http://dx.doi.org/10.17582/journal.aavs/2021/9.12.2234.2246
ISSN (Online) | 2307-8316; ISSN (Print) | 2309-3331
Copyright © 2021 Abdelftah et al. This is an open access article distributed under the Creative Commons Attribution License, which permits unrestricted use, distribution, and reproduction in any medium, provided the original work is properly cited.
INTRODUCTION
Optically, the cornea is considered the eye’s transparent, the anterior layer protecting the eye and refracting light. It is coated with a tear film that offers nearly two-thirds of the refractive power of the eye together with the underlying tissue (Nejad et al., 2015). The cornea is made up of five layers in many mammals. Whereas their arrangement from the outer to inner is the epithelium, Bowman’s membrane, the stroma, Descemet’s membrane, and the endothelium (DelMonte and Kim, 2011).
Considering, the epithelium is the cornea’s outermost layer with regeneration capability after an injury. Furthermore, the endothelium is made up of non-renewable cells that maintain the dehydrated stroma and transparent cornea. The endothelium’s permeability can influence the cornea’s transparency and capacity to work properly (Iannaccone et al., 2012). The stromal collagen fibril arrangements play a significant part in cornea’s mechanical strength. Throughout the lamellae, the permanent orientations of the collagen fibrils lead to corneal anisotropy (Sheppard et al., 2010). In addition, the keratocytes connect to their adjacent cells by interconnecting fine cell procedures and constitute cellular networks (Murata et al., 2019). Varieties in corneal epithelium thickness considered a structural adaptation occurring in different vertebrates and allowing them to adapt to a broad range of environmental circumstances (Edelhauser, 2006; Hutcheon et al., 2019).
Many authors have shown in mammals that there are a decrease in corneal metabolism and decrease in the glycogen deposition, besides adversely impacts the barrier characteristics and transport functions of both epithelium and endothelium, leading to an rise in corneal thickness and a loss of transparency (Edelhauser, 2006; Parekh et al., 2019). Metabolic power and glycogen energy are crucial for the maintenance of mammalian corneal border layers ‘ barrier function and ionic pumps, especially corneal endothelium. Furthermore, there are decrease in epithelial corneal density , therefore the endothelial cells develop tight junctions. Also, theendothelial permeability decreases, the number of endothelial cell pump sites and density increase, consequently the total corneal hydration decrease resulting in a transparent cornea (Edelhauser, 2006). Comparative morphological surveys have recognized a variety of animal eyes, including pinhole; mirror and single-lens camera eyes by several techniques (Land and Nilsson, 2012). On the other hand, there are little investigations on some species of mammals like bats. Besides, the present study aims to correlate between the significant effect of the environmental condition, and the morphological and anatomical structures of the cornea in the investigated species.
MATERIALS AND METHODS
Experimental animals
Three mammalian families of the present study were collected from different locations of Egypt and were identified according reviews of Mammalian (Saleh, 1997). Ten animals from each family were used in the present study. The animals were kept under observation for about 15 days before the onset of the experiment to exclude any intercurrent infections and to acclimatize on the laboratory conditions. Their common name, distribution and their habitat were explained in the present investigation.
Family pteropodidae: Rousttus egyptiacus (E. Geoffroy, 1810)
Rousttus egyptiacus (E. Geoffroy, 1810) has the common name as Egyptian fruit bat. They distributed in Behira Governornate, Egypt (30.8481° N, 30.3436° E) and Abo- Rwash (30.0131° N, 31.2089° E). They were found in moist tropical habitat and subtropical areas. They considered as nocturnal animals and spent their days roosting in trees or caves, often with large groups of other bats with weight ranged 980± 260 g (Mickleburgh et al., 1992).
Family leporidae: Oryctolagus caniculus (Linnaeus, 1758)
Oryctolagus caniculus (Linnaeus, 1758) has the common name as the Domestic rabbit. They distributed Behira Governornate, Egypt (30.8481° N, 30.3436° E) and Abo- Rwash (30.0131° N, 31.2089° E). They preferred a mixed habitat of Mediterranean oak savanna, or area with 40% cover for shelter from predators. They considered as herbivorous mammals and gregarious and readily domestics. They were being most active around dawn and dusk, although they weren’t infrequently seen active during the day 1120±320g (Lombardi et al., 2003).
Family muridae: Rattus norvegicus (Berkenhout, J. 1769)
Rattus norvegicus (Berkenhout, J. 1769) have common name as Albino rat. They distributed Behira Governornate, Egypt (30.8481° N, 30.3436° E) and Abo- Rwash (30.0131° N, 31.2089° E). They inhabited sandy and sometimes rocky areas and live in tunnels under shrubs with diurnal activity with weight ranged from 120±12.0g.
Experimental methods
After enucleation the corneas were dissected free from the orbit with a sharp out razor blade and prepared for histological, histochemical and scanning electron architectures as follows:
Histological sections
The fixed specimens of the cornea were washed to remove the excess of the used fixative. They were then dehydrated in ascending grades of ethyl alcohol 70, 80, 90 and 95% for 45 minutes each, then in two changes of absolute ethyl alcohol each for 30 minutes. This step was followed by clearing in two changes of xylene, each for 30 minutes. The tissues were then impregnated with paraplast plus (three changes) at 60oC for three hours and then embedded in paraplast plus. Sections of 4 to 5 µm thickness were prepared with a microtome and stained with hematoxylin and eosin for histopathological examination (Suvarna and Layton 2013). After light examination for the corneal histological sections; the presence and absence of different layers were recorded as follows: absent (-); one layer (+); two layers (++) and three layers (+++).
Histochemical sections by Periodic acid-Schiff’s (Passo et al., 2019)
The fixed specimens de-waxicated by xylene and rehydrated through graded ethanol to water. Then they oxidized with 1% aqueous periodic acid solution (H5IO6; WINLAB; Leicestershire, UK) for five minutes. After that, they were rinsed in distilled water and were covered with Schiff’s reagent for 10-15 minutes then rinsed in running tap water for five minutes. Finally, the specimens were dehydrated, clear with xylene, mounting, and covered slips. The sections were ready for examining by light microscope (McManus, 1946; Bancroft et al. 2013). The degree of carbohydrates was evaluated and represented as - = absence; + = mild; ++ = moderate and +++ = high.
Scanning electron microscopy (SEM)
The whole eye was immediately fixed overnigt with modified Karnovsky solution (2% paraformaldehyde and 2.5% glutaraldehyde containing 0.1 M cacodylate-buffer, pH 7.4) according to Morris (1965). The fixed specimens were then washed in 0.1 M cacodylate buffer and post-fixed in a cacodylate-buffered solution of 1% osmium tetroxide at 37°C for 2 hours. The corneal specimens were rinsed in increasing concentrations of ethanol to commence dehydration which the first rinsed in 50 % ethanol for 5 minutes; then 3 times for 5 min with 70% ethanol; rinsed 3 times for 5 min with 90% ethanol, rinsed 2 times for 5 min in 100 % ethanol; and finally rinsed 2 times for 10 minute in 100% ethanol (molecular sieve) all ethanol was removed from the samples and replaced with hexamethyldisilane (HMDS) in fume hood and left for 10 minutes. The base of the metal SEM stubs was labeled and applied double-sided conductive carbon tape to the top of the stubs. The dried specimens were then sputtered with gold in Joel fine coat Ion Sputter (SPI-Module). Specimens were then examined and photographed using standard microscope operating procedures JEOL SEM (JSM-5400 LV) for visualization of specimens in the Microanalysis Centre, Faculty of Science, Beni-Suef University, Beni-Suef Egypt. The analysis was commenced at low magnification, then the magnification increased gradually at an accelerating voltage of 15kv (JSM.5400LV, JEOL) (Jeffree and Read 1991; Goldstein et al., 2017).
Image analysis
The anterior surface of the investigated cornea of each studied species was examined by JEOL (JSM with accelerating voltage 5400 LV). The area of the individual epithelial cells (µm2) and the number of cells were measured (Abràmoff et al., 2004). Moreover, the mean of epithelial cell density was calculated in addition to the diameters of both microholes and blebs. These image analyses were digitally recorded using Image J software.
Statistical analysis
Data were analyzed using one- way analysis of variance (ANOVA). Mean for ten readings for the corneal surface for each parameter (microholes and blebs) at least for measuring. Data were expressed as mean ± standard deviation (SD). Values of P> 0.05 were considered statistically non-significant, while values of P< 0.05 were considered statistically significant.
Ethics committee approval
All animal procedures were conducted following the standards set in the guidelines for the care and use of experimental animals by the Animal Ethics Committee of the Zoology Department in the Faculty of Science at Beni-Suef University (under an approval number is BSU/FS/2015/9.
RESULTS and Discussion
Histological observations
The cornea of Rousttus egyptiacus, Oryctolagus canuculus, and Rattus norvigcus are illustrated throughout Figure 1. They arranged as a superficial epithelial layer, followed by stroma and ended with the endothelium layer. Stromal lamellae were characterized by good distribution of keratocytes in its matrix. Besides, their cornea ended with monolayer of endothelium of flat squamous cells. Bowman’s membrane was illustrated in R. egyptiacus and O. canuculus, but absent in R. norvigcus. Descemet’s membrane appeared only in O. canuculus. The interfibrille space spread only in R. egyptiacus, O. canuculus among their collagen lamellae (Figure 1 and Table 1).

Figure 1: Photomicrograph of transverse section of the cornea of Rousttus egyptiacus (a, b) Oryctolagus cunculus (c, d), Rattus norvegrious (e, f). (a, c, e H and E X100). (b, d, f H and E X400).
Particularly, the epithelial layer of Rousttus egyptiacus was distinguished by one layer of basal columnar cells, followed by six rows of polyhedral cells and ended with one row of flat squamous cells. However, cornea of O. canuculus, their epithelium based on one row of cuboidal cells with round nucleus, four rows of polygonal cells and ended with one layer of flat squamous cells. On the other hand, R. norvigcus cornea was distinguished by a simple epithelial layer which arranged as one layer of basal cuboidal cells, then another one layer of polygonal cells and ended with flat squamous cells (Figure 1 and Table 1).
Table 1: Comparison between corneal layers and its histological structure in three species of mammals:
Layer |
Epithelial layer (Types of Cells / Layers) |
Bowman’s membrane | Stroma | Desecmet’s membrane | Endothelium | ||||
Mammals | Basal columnar layer | Basal cuboidal layer | Polyhedral layers | Flat squamous layer | |||||
Rousttus egyptiacus | + | - | ++++++ | + | + | + | - | + | |
Oryctolagus caniculus | - | + | ++++ | + | + | + | + | + | |
Rattus norvigus | - | + | + | + | - | + | - | + |
Table 2: Comparison between the thicknesses (Mean ±SD) of corneal layers in three species of mammals (10 animals per species). Layer’s thickness that are not sharing common superscripts denote significant differences (P< 0.05).
Species |
Total thickness (µm) |
Corneal Layers | ||
Epithelium thickness (µm) (%) |
Stroma thickness (µm) (%) |
Endothelium thickness (µm) (%) | ||
Rousttus egyptiacus |
340.7±24.9b |
35.7±4.3a (10.4%) |
301.0±38.6b (88.5%) |
3.9±0.2a (1.1%) |
Oryctolagus caniculus |
480.9±104.2c |
33.3±3.9a (7%) |
441.6±54.6c (91.8%) |
6.2±1.3b (1.2%) |
Rattus norvigus |
281.0±46.6a |
37.2±8.4a (13.4%) |
221.2±20.6a (78.7%) |
26.4±4.8c (9.3%) |
F Valueou | 34.1 | 2.6 | 42.8 | 339.5 |
P Value | 0.000 | 0.08 | 0.000 | 0.0000 |
Obviously, the thickness of the mammalian epithelial cornea showed non- significant relation between the examined mammalians’ species (P<0.05) whereas, Table 2 recorded 35.7±4.3, 33.3±3.9 and 37.2±8.4 µm for R. egyptiacus, O. caniculus and R. norvigus respectively. Therefore, this data revealed that the mammalian epithelium constituted relatively 10% of corneal thickness.
Furthermore, the stromal thickness of the studied species apparently constitued around 86% from the total thickness. Table 2 showed a statistically significant relationship among the investigated species (P<0.05), which was recorded in R. egyptiacus and O. caniculus and R. norvigus 301.0±38.6, 441.6±54.6 and 221.2±20.6 µm respectively.
Moreover, the endothelial thickness was measured in R. egyptiacus, O. caniculus and R. norvigus 3.9±0.2µm, 6.2±1.3µm and 26.4±4.8 µm respectively and reflect a highly significant relationship among the investigated species (P>0.05). Table 2 revealed that the endothelium thickness registered around 1% from the total thickness except R. norvigus’s endothelium, which reported relatively 9% from the whole thickness.
The current investigation revealed that the highest total corneal thickness and the stromal layer (91.8%) recording for O. caniculus. In contrast to R. norvigus has the higher epithelium and endothelium layers 13.4% and 9.3%, respectively.
Histochemical observations: (Periodic acid Schiff (PAS) reaction)
Generally, the large storage of glycogen in corneal epithelium plays a vital role in the transparency function of cornea. Glycogen distributed among the corneal layers of the investigated vertebrate’s species. Epithelium and endothelium are richer in glycogen than the stromal layers in some species. Bowman’s and Descemet’s membranes were represented in some species and others were not.
The epithelial layer of the mammalian cornea was intensely stained in Rattus norvigus however, it moderated in Oryctolagus cunculus and Rousttus egyptiacus. On the other hand, stroma and endothelium layer had strong PAS reaction in all studied species Rousttus egyptiacus, Oryctolagus cunculus and Rattus norvigus, respectively. Concerning Bowman’s membrane had strong affinity to PAS materials in only two examined species (R. egyptiacus and O. caniculus). However, Descemet’s membrane is completely absent from the three investigated species (Figure 2 and Table 3). It was noticed that the basal columnar of the epithelium possessed high affinity to PAS materials.
Scanning electron microscopy (SEM) observations
The mammalian central cornea distinguished by highly significance cell density, which R. egyptiacus had the highest value 8428.0±450.4cells per mm2, while R. norvigus recorded 2693.4±308.7cells per mm2 and O, caniculus had 2402.9±178.8cells per mm2 (Table 4).
Irregular polygonal epithelial cells were mostly predominantly in two mammalian investigated species O, caniculus and R. norvigus, although it was regular in shape in cornea of R. egyptiacus. Concerning, microplicae which considered as surface micro projection and were exposed by two types of polymorphic cell according to their scatter electron in each examined species which was light (L) and dark in R. egyptiacus and O, caniculus, however medium and light in R. norvigus.

Figure 2: Light micrograph of transverse section of the cornea of Rousttus egyptiacus (a, b) Oryctolagus cunculus (c, d), Rattus norvegrious (e, f). Showing PAS reaction in the corneal layers. (a, c, e): (PAS X100), (b, d, f): higher magnification (PAS X400).
The light type was represented by dense microplicae covering the surface of light cells, where the medium cells formed of semi dark cells covered with moderate density of microplicae, while dark ones consisted of dark epithelial cells, which had less density of microplicae (Figure 3). Microprojection was represented by various diameter over the corneal surface of O, caniculus and R. norvigus. In spite of blebs distributed on the surface of R. egyptiacus in round shapes and with different diameters 5.8±1.6µm (Figure 4 and Table 4).
Concerning the histological examination of the studied mammalian corneal sections referred to presence of five morphological layers; Defensor epithelium, mechanical barrier basement membrane Bowman’s membrane, hypocellular stroma, bounded Descemet’s membrane and integrated endothelium. Similarly, these observations are postulated (El-Dawi, 2005) in some mammalian species.
The current results referred that the mammalian corneal transverse sections composed of stratified squamous epithelial layer in the three investigated species. (Doughty, 1990; Brookes, 2018) confirm the same findings in rabbits and rats.
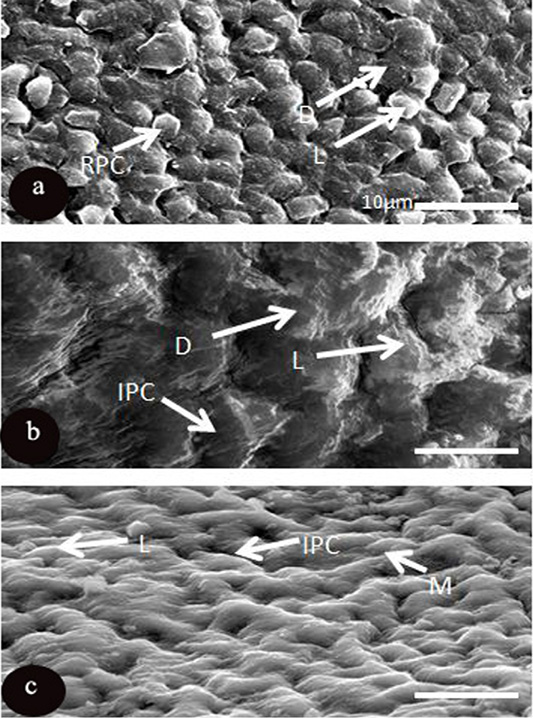
Figure 3: SEM micrograph of the corneal epithelial cell of three mammalian species a: Rousttus egyptiacus b: Oryctolagus cunculus; c: Rattus norvegrious. (Scale bar, 10μm).
Optically, it is noticed that the increase in number of epithelial layers protect the cornea from any chemical or mechanical abrasion and stress from the outside environment. Similar observations are suggested in Rattus rattus and Meriones shawi (El-Dawi, 2005) and in the Knockout mice (Doughty and Fong 1992; Chakravarti, 2001; Saadi-Brenkia et al., 2018). Unlike (Gibson et al., 1995; El‐Bakry 2011) are in disagreement with our findings in some species of mammals.
Table 3: Comparison between three investigated mammalian species according to carbohydrate content in cornea by using Periodic Acid Schiff (PAS) stain: (+++) strong reaction; (++) moderate reaction; (+) weak reaction; (-) absent Periodic Acid Schiff (PAS) stain.
Mammalian | Epithelium | Bowman’s membrane | Stroma | Descmet's membrane | Endothelium |
Rousttus egyptiacus |
++ | +++ | +++ | - | +++ |
Oryctolagus cunculus |
++ | +++ | +++ | _ | +++ |
Rattus norvigus | +++ | - | +++ | _ | +++ |
Table 4: Comparison between the cell density (Mean ±SD) of corneal epithelial layer and diameter of blebs and microholes in three mammalian species (10 animals per species). Measurements that not sharing common superscripts denote significant differences (P<0.05). NM: not measured. * (NM), not measured. All measures of epithelial cell density are calculated from central cornea.
Class | Species |
Epithelial cell density (cells/ mm2) |
Blebs (µm) | Micro holes (µm) |
Mammals | Rousttus egyptiacus |
8428.0±450.4b |
5.8±1.6 | NM* |
Oryctolagus caniculus |
2402.9±178.8a |
NM* | NM* | |
Rattus norvigus |
2693.4±308.7a |
NM* | NM* | |
F value |
629.2 | NM* | NM* | |
P | 0.000 | NM* | NM* |
Accordingly, taking into consideration that ocular size affects the number of epithelial layers, as a response to adapt with the hard conditions of their terrestrial environments. These considerations probably confirmed the correlations between the optical size and increase in thickness of the epithelial layers thus, leading to the formation of an excellent focal system. That might be a monofocal system such as in Canis lupus lupus and Canis lupus familiaris or multifocal optical system as in Mus musculus (Malmström and Kröger 2006; Neagu and Petraru, 2015). The morphological function of the epithelial layer of the studied species reflects its physiological activities such as biochemical reaction, refraction power and accommodation with hard habitat. Variation of environmental agents among examined species like nocturnal vision in Rousttus egyptiacus and diurnal vision in Oryctolagus caniculus and Rattus norvigus reflect the specialization of the morphological design of the nocturnal cornea and its strong curving to provide higher refractive index, that estimated entrance of more light to the retina for improving the transparency of cornea and adaptation with the nocturnal vision. These architectural designs are found in some nocturnal mammals (Neagu and Petraru, 2015)
Such forested habitat of R. egyptiacus rather than the other two investigated species, multi polygonal layers arranged in its epithelial layer as a mechanical defense and accommodation mechanism with nocturnal conditions against any environmental influences. Similar recordings is addressed in nocturnal species of Bubo Bubo africans (Pourlis, 2013).
Another characteristic structure in the examined mammalian corneal sections is uniformed distribution of condensed collagen fibrils. They arrange as one lamellar zone without interpretations and with relatively equal interfibrillar spaces. Such unique architecture is reported in some land mammals (Lemp et al., 1970; Leonard et al., 2016). In vitro studies of (Nagayasu et al., 2009; de Oliveir et al., 2018) refused our findings on other mammals such as dogs and also (El-Dawi, 2005) in Rattus rattus and Meriones shawi.

Figure 4: SEM micrograph of the corneal epithelial cell of three mammalian species showing. a: Rousttus egyptiacus; b: Oryctolagus cunculus; c: Rattus norvegrious. Note: blebs (Bb) and microprojection (MPJ) (Scale bar, 20μm).
It is noticed that the collagen lamellae of nocturnal studied mammals (R. egyptiacus) have relatively large interfibrillar spaces than other two diurnal examined species (O. caniculus and R. norvigus) which assist in the formation of a clear and sharp retinal image. As large interfibrillar spaces allow a large image to reach to the retina and enhancing the corneal transparency (Meek and Knupp, 2015; Neagu and Petraru, 2015).
To date, many attempts to explain the correlations between the corneal transparency and the complexity of the collagen lamellae whereas the transparency increases with decreasing the parallelism and condensation of the stromal lamellae (Meek and Knupp, 2015). So, these findings are suggested in our investigated species, with relative appearance of the loose lamellae in R. egyptiacus, more condensed in O. caniculus and most condensed in R. norvigus.
As mentioned in the current histological examination, uniformed distribution and diameter of the collagen fibril of the stromal layer played a vital role in the corneal transparency (Meek and Knupp, 2015).
Excellent hexagonal lattice was constricted from interconnecting of the fibrils with each other, preserving distance between them. Similarly, the intensity of the entering light is inversely proportional with the radius of the fibrils. The present data confirmed these findings in the examined species, in which R. egyptiacus possessed the lower fibril radius and the higher interfibrillar space, therefore its corneal transparency recorded the highest level, as a response to its nocturnal habitat.
Regarding the arrangement of collagen fibrils, (Chakravarti et al., 1998; Chen and Birk, 2013) proposed the ultimate repulsion forces exist between the dispersed collagen lamellae to prevent corneal opacity and providing transparency. In view of these findings, it seems relative that corneal stroma might be responsible for corneal transparency and formation of sharp retinal. Therefore, any disturbance in the uniform interfibrillar spaces caused loss of transparency. These findings came out in agreement with bovine cornea (Freund et al., 1995; Nishida et al., 1997; Strandberg, 2015; Ling and Bastion, 2018).
It is noticed that moderate density of keratocytes sparsely distributed on the stromal matrix of the mammalian investigated species, was considered as a transparent object except their nuclei. Obviously, there are regular dispersions of keratoyctes (small spindle shaped cells), whereas constricted to the anterior portion of the stromal layer with a few densities in case of R. egyptiacus. Also, it was uniformly distributed over the whole layer with moderate density in O. caniculus and spreading over the stromal of R. norvigus with high intensity. Relative distribution is found in rabbits, humans and other different mammals (Lemp et al., 1970; Leonard et al., 2016).
Construction of collagen molecules and proteoglycans is the main task of keratocytes, besides formation of matrix metalloproteinases (Freund et al., 1995; Meek and Knupp, 2015). Furthermore, acting as transient cells, which activated as macrophage or dendritic cells in case of injury, generating myofibroblasts precursors. Hassell and Birk (2010) observed the same findings in bovine cornea. These justifications supported our results in R. norvigus that enables its cornea to accommodate with the highest activity in a hard habitat.
Currently, this study described the keratocytes as light scatter as they are able to change the refractive index for any cytoplasmic alterations; therefore, they increase the corneal opacity and decrease clear transparency. This explains the low density of keratocytes in the nocturnal R. egyptiacus. The present findings came in accordance and parallel other land mammals (Jester et al., 1999; Møller-Pedersen, 2004).
Interestingly, only two of the cornea of present mammalian species (R. egyptiacus and O. caniculus), composed of Bowman’s membrane with relatively small thickness, that is considered as a bounded basement membrane, which separates the epithelial layer from the collagen lamellae of the stroma. Besides it appeared as lusterless without any internal structure, however it is identified as a homogenous and excellent transparent elastic Lumina. It was previously reported that the Bowman’s membrane is thinner in lower mammals such as rodents and rabbits (Hayashi et al., 2002) and become thicker in higher mammals like human. Similar observation was confirmed in our investigations of O. caniculus (lower mammals) and camel and guinea pig (higher mammals) (Merindano, 2002)
Almubrad and Akhtar (2011) confirmed this result in mice, rats, rabbits, cattle, tree shrew and humans. They added that the variation in thickness of the Bowman’s membrane might be due to the fixative substance. Furthermore, other histological investigations e.g. Merindano (2002); Hayashi et al. (2002) and Svaldenienė et al. (2003) came in agreement with the same data in higher mammals such as dogs, cattle, human, mouse, rat, guinea pig, rabbit, cat and most carnivores’ animals. In contrast to these observations, results of many authors e.g. Ohji et al. (1994); Gibson et al. (1995); Krachmer et al. (1997); El-Dawi, (2005) and Miao et al. (2019) disagree in other mammalian species Rattus rattus and Meriones shawi.
Its contributions appear in preserving the corneal curvature; rigidity and stiffness. As for accommodation, it is responsible for increasing the refracting power of the cornea, by increasing the corneal curvature to bring the far object closer. Such variation in habitats of the investigated species aroused from opposite light conditions (nocturnal and diurnal habitat) and reflect on the light sensitivity of the eye, morphological structure of cornea and physiological properties of the retina (Larson, 2004; Jones et al., 2007; Rosencrans, et al., 2018).
Taking into consideration the accommodation range of the flattened cornea, the Bowman’s membrane greatly affects in increasing the light sensitivity of nocturnal R. egyptiacus more than diurnal R. norvigus (which lacks it) and increasing its refracting power, as the vision process estimates the mating process, hiding from enemies and aposematism of the vision. Justification of these findings reported in mouse, rat, guinea pig, rabbit and cattle (Larson, 2004; Rosencrans et al., 2018).
Indeed, there is an optical and physiological correlation between the presence of Bowman’s membrane and absorptions of UV, as it acted as an effective filter of UV- radiation with its unique constriction through its arrangement of the collagen fibrils and its molecular composition, to protect the internal structure of the glop from excessive radiation. Furthermore, its absorption coefficient is eight times higher than the coefficient of the stromal layer (Kolozsvári et al., 2002) and (Khan et al., 2019). These explanations illustrated the presence of Bowman’s membrane in the cornea of the investigated O. caniculius species which inhabited in diurnal conditions. Similar observations reported in higher mammals such as cattle, cat and pig (Hayashi et al., 2002).
The microscopic level referred to the Descemet’s membrane observed only in O. caniculus, as a specialized basement membrane that separates the substenia propria from cellular endothelium layers with an amorphous appearance in the form of meshwork of homogeneous fibrils. This parallelism was identified in cattle and rabbits (Hayashi et al., 2002).
Obviously, it represented a secretion of the endothelial layer which had integrated function and preserving the corneal stiffness. Similar observations recorded in camel and bovine (Massoudi et al., 2016). As a consequence of the preservations of the morphological features and the integrated function of the endothelium in various physiological conditions, Descemet’s membrane acts as a molecular barrier that prevents the infiltration of certain proteins from the aqueous humor into the corneal stroma (Swash and Still, 2005; Chen et al., 2017). The corneal endothelium of three examined species was represented as monolayer of flat squamous epithelial cells. These observations came out in agreement with various vertebrate groups, such as amphibians, reptiles, birds and mammals (Yee et al., 1987; El-Dawi, 2004).
Maintenance of corneal transparency and the integrity of corneal layers depend on the morphological and physiological healthy of the endothelium. A major task of the endothelium is stromal dehydration and maintaining sodium and potassium ions by an active sodium-potassium pump (Neagu and Petraru, 2015)
Furthermore, it acted as a leaky barrier that transports some ions and macromolecules between nutrient rich aqueous humor and stromal layer against a concentration gradient (Edelhauser, 2006). As mentioned in the previous data, the specialized endothelium has many activated traits, because they have a large number of mitochondria in their cytoplasm, which allows this tissue to be engaged in fluid transportations (Akhtar et al., 2008).
Endothelial population cells that are influenced by age and lesions result in increasing corneal thickness and hydration of the corneal stroma through fluid filtration from the aqueous humor in monkeys, horses, dogs (Tamayo-Arango et al., 2009). Therefore, alterations in the physiological properties of this layer causes corneal opacity and edema.
The high variability in the significance of statistical analysis of corneal thickness in present mammalian species, reflect accommodation with harsh surrounding conditions and various ecological life style (McFarland, 1991). As mentioned in the present study, O. caniculus recorded the highest corneal thickness among the studied species.
The present findings reported that the rabbit cornea 480.9 µm which is thicker than frog 80 µm and thinner than of the sheep 600 µm. Therefore, some studies addressed that corneal thickness is directly proportional with the body weight and size (Scott and Bosworth, 1990; Saadi-Brenkia et al., 2018). Besides, Tamayo-Arango et al. (2009) indicated that higher corneal thickness revealed to a large amount of the collagen matrix in the thicker stromal layer that is required for high metabolic activity of the cornea. Another histological explanation was referred that the increase in corneal thickness seems likely to increase the amount of collagen in the stromal layer.
Nevertheless, all corneal layers should be transparent with minimal light scattering property, to achieve the dual function of the cornea. It provides more than of 30% of the refractive power of the eye, and protects the underlying layers of the eye (Meek and Knupp, 2015). Consequently, micro anatomical analysis showed that, the epithelium constitutes relatively about 10% from the corneal thickness. The present study confirmed these findings, in R. egyptiacus 10.4% (Reinstein et al., 2008), mouse 11.1% and in cattle 12.5%. These explained that the epithelial layers of the three examined species have non-significant variance (P>0.05), whereas Lemp et al. (1970) confirm that result between some mammalian species.
On the other hand, R. norvigus had the higher thickness of the two cellular layers (epithelium 13.4% and endothelium 9.3%) as a response to the protection of the rest layers of the eye and preventing the stromal hydration to reduce the corneal opacity and stimulate the corneal transparency. These observations came in accordance and parallel to Hayashi et al. (2002) in most of carnivores species and monkeys.
Regarding the affinity of the mammalian corneal layers to PAS stain, it is observed that the all corneal layers of R. norvigus appeared strongly stained. In contrast to other, two investigated species (R. egyptiacus and O. caniculus), whereas they reported moderate affinity only in the epithelial layer and a strong reaction with PAS stain in the stromal and endothelium layers. Consequently, Bowman’s membrane appeared strongly stained in two investigated species (R. egyptiacus and O. caniculus). On the other hand, Desecmet’s membrane was completely absent in the three investigated species.
Obviously, the rate of the metabolic activity in the cornea is directly proportional with the corneal glycogen content (Tamayo-Arango et al., 2009). Therefore, the cellular epithelium and endothelium are highly metabolically activated due to their aerobic glycolysis and oxygen consumption, besides the stromal layer considered as active as the two cellular layers due to its abundance from the polysaccharides ground matrix (Kinoshita, 1962). This data is consistent with the present observations, and explains the high affinity to PAS reaction in corneal layers of R. norvigus. Furthermore, type of habitat and sort of highly activated conditions of this species has an impact on its metabolism and its aerobic glycolysis, besides its molecular organizations of compacted collagen lamellae and its high polysaccharides content ground matrix. Such structure is similar somewhat to that described in diurnal desert rodent Psammomys obesus (Saadi-Brenkia et al., 2018).
At the molecular level, declining of the corneal opacity and cloudy features result from the homogeneity of all constituents of the cornea (Meek and Knupp, 2015). This data is ultimately related to the histological composition, life style and the metabolic activity of the cornea, whereas the deficiency in the variability of the epithelial layers of two investigated species (R. egyptiacus and O. caniculus) emphasize the moderate affinity to PAS stain and stimulation of the homogeneity of the epithelial layer, resulting in corneal transparency. Moreover, the current results confirmed with others in rats, dogs, rabbits and humans (Meek, 2008).
Accommodation in peculiar habitats of the investigated species frequently stimulated the anatomical modifications of the corneal layers especially in the stromal and endothelial layers, which enables species’ cornea to adapt with their typical ecological surroundings (Saadi-Brenkia et al., 2018). Nocturnal R. egyptiacus possess molecular adaptations by accumulating glycogen in the endothelial layer to supply its high metabolic activity in making the stroma in dehydration state, besides presence of large interfibrillar spaces between its collagen lamellae which in turn improve its corneal transparency. These findings are in accordance with (Regini et al., 2004; Meek, 2008) in the endothelial layers of cattle and mice cornea.
Despite the stromal layers of diurnal O. caniculus packed with glycogen content, which are responsible for supporting the corneal metabolism and its aerobic glycolysis to fit the activation of its large body weight. Currently, high affinity of Bowman’s membrane to PAS stain in O. caniculius and R. egyptiacus referred to molecular organization of elastic lamina (connective tissue packed with glycogen) in the Bowman’s membrane. These interpretations and correlations between the content of polysaccharides and the histological compositions referred to highly adaptive range of the investigated species.
The image analysis data revealed that the mammalian cornea of the examined species showed significant variability in their cell density (P<0.000), whereas R. egyptiacus possessed the higher statistical variance than other two species. As the current findings indicated that the corneal transparency strongly correlated with the epithelial cell density, besides the corneal thickness, whereas the increasing cell density especially in the epithelial layer, increase the chance in improving the corneal transparency and reducing the corneal cloudy. These findings were consistent with many authors e.g. Arndt, (2001) and Svaldenienė et al. (2003) in dogs and pigs.
Concerning the characteristic features of pleomorphism of the representative examined species that reported a high degree of polygonality in the form of regular polygonal epithelial cell in R. egyptiacus and R. norvigus, but irregular polygonal cells in O. caniculus with distinctly border-like cell breaks. Most probably, the same results reported in cats, monkeys and pigs (Tamayo-Arango et al., 2009)
Pleomorphism traits share in the protection of the corneal epithelium and stabilizing the tear film (Collin and Collin, 2006; El‐Bakry, 2011). Microstructure of the corneal surface demonstrated the correlation between the different ecological surroundings that ranges from the forested habitats (O. caniculus and R. egyptiacus) to desert arid areas (R. norvigus) and various visual conditions (diurnal and nocturnal). Variability in the refractive indices of the corneal epithelial cells when the air surrounded the corneal surfaces (terrestrial species) reflect on the pleomorphism which in turn increase the refractive power of the cornea through increasing the intensity of the reflected light on the retina (Collin and Collin, 2006). Based on these observations, the current study proposed that the increasing of corneal transparency in cornea of nocturnal R. egyptiacus result from its higher pleomorphism and their cell density.
Microtopography of the mammalian cornea distinguished by spreading of microplicae on the corneal surface that sorted into three types light and dark in R. egyptiacus and O. caniculus and medium and light in R. norvigus. Previous attempts tried to resolve presence of microplicae that might be result from various microchanges on the corneal surface, as accommodated response with the different environmental conditions. They developed as precursor of microprojection (microvilli or microridges) on the corneal surface of cat, mouse, sheep (Ovis aries) and rabbit (Pfister and science, 1973; Collin and Collin, 2006).
Various explanations that elucidated three types of microplicae could be sorted into light and medium and dark, that might be classified according to their scattering electron on basis of the quantity of microprojections, whereas the light type brings dense microprojection, medium cells covered by moderated microprojections and dark ones have few microprojections (El‐Bakry, 2011). These microprojections can be identified based on the maturation of the cell, however at the pre mature phase appear light and also the post metamorphic stage they appeared dark (Pfister and science, 1973; Collin and Collin, 2006). These characteristics to possess high quantity of ascorbic acid in the dark cells to absorb UV- harmful radiations. Alongside, medium cells have a moderate affinity to ascorbic acids, as well as the light cells have a little amount of the acid (Massoudi et al., 2016; Khan et al., 2019).
It is obviously that the cornea of individual investigated species might possess more than one microstructural adaptation trait to preserve their accommodation range. In particular, presence of microplicae is considered a dynamic requirement for healthy habitat preserving stable structure besides, it can change under certain conditions. These observations came in accordance and parallel to that reported in rabbit cornea. The latter case changes from regular erect microplicae in normal conditions to short irregular microplicae, when incubating at various bicarbonate solutions (Doughty, 1990; Khan et al., 2019).
The present study does not recognize any microholes on the corneal surface of the three investigated species. In contrast, previous data identified microholes on the corneal surface of sheep (Ovis aries) and Australian kolola (Doughty and Fong, 1992) or represented site of increasing membrane bound vesicles into tear film for stabilizing its structure. It’s well known that the main integrated functions of the microholes are maintaining lubrication of the corneal surface for protection from the high osmolarity medium (aquatic species) and high evaporated habitat (terrestrial species live in high temperature), and reducing fractional rate of the cornea to adapt with quick movement of ocular adnexa (Hayashi et al., 2002). Due to absence of these extreme habitats in the present studied species, microholes did not appear on the epithelial surface. Collin and Collin (2006) referred the absence of the microholes from the mammalian vertebrates to its independent evolution in response to amphibious and burrowing habitats.
Another characteristic feature was noticed, dispersion of the microprojections over the corneal surface of only two investigated species (R. norvigus and O. caniculus) with high variability in diameters and shapes. On the other hand, corneal surface of R. egptiacus possess blebs (regular spherical microprojections with various diameter). The recent preliminary data of Islam et al. (2019) showed that the design of microprojections is influenced by ocular adnexa like presence or absence of the eyelids and nictating membrane and also by necessity for lubrication and reducing the fraction.
Similar investigations on the cornea of R. rattus and M. shawi showed that the surface bulged with various diameter blebs, which contributed in the mitotic activity of the epithelium and greatly influenced by direct exposure to extremely surrounding factors of its habitat.
The microprojections and blebs on the investigated corneal surface aid in increasing the surface area for movement of water and metabolic products through cell membrane. In addition, they improve the oxygen consumption and nutrients, which in turn improving the corneal activation of diurnal R. norvigus and O. caniculus. Also, they can protect the underlying optical layers, and elevated the level of the corneal transparency of the nocturnal R. egyptiacus, and as in case of some mammals e.g. cornea of rabbits, cats and pigs (Doughty, 1990, 1991).
Not only the microprojections stabilized the tear film, but also contributed in the refractive power properties which their diversity of patterns stimulating the light capture and increasing the intensity of the light entrance, therefore improving the corneal transparency and preventing corneal opacity.
CONCLUSIONS AND RECOMMENDATIONS
Various habitats of the investigated species had various significant effects on the histological, histochemical and ultrastructural level of the cornea to accommodate to different environmental conditions. The acquired data will increase our understanding of the environmental constraints placed on the reptilian cornea and add to our knowledge of the evolutionary development of this tissue. Moreover, to our knowledge, the present study is considered the first to evaluate corneal microstructure in Egyptian’s Bats.
Author’s Contribution
All authors contributed equally.
Abbreviations
Epithelium (EP), Stroma (S) and Endothelium (EN), Keratocytes (K), Squamous cell (Sq), Basal columnar cell (Bc), Polyhedral cell (Py), Bowman′s membrane (Bm) and Interfibiller space (IS), Descemet′s membrane (DM), Basal cells of epithelial layer (Bc), Regular polygonal epithelial cells (RPC), Irregular polygonal cell (IPC), Light type (L) and Medium type (M) and Dark type (D), Blebs (Bb) and Microprojection (MPJ).
Conflicts of interest
The authors have declared no conflict of interest.
REFERENCES