Advances in Animal and Veterinary Sciences
Research Article
Preparation, Characterization and Antibacterial Activity of Chitosan Nanoparticle and Chitosan-propolis Nanocomposite
Sawsan M.A. El-Sheikh1, Abd El-Alim F. Abd El-Alim1, Hosny Abd El-Fadil Ibrahim1, Elham A. Mobarez2, Dalia M.A. El-Masry2, Walaa A. El-Sayed3
1Pharmacology Department, Faculty of Veterinary Medicine, Zagazig University, 44511, Egypt; 2Animal Health Research Institute, Dokki, Egypt; 3Animal Health Research Institute, Zagazig branch, 44516, Egypt.
Abstract | Bacterial resistance and antibiotics residues due to over and misuse of antibiotics need to overcome through formulation of novel natural-based nano antimicrobial agents. Propolis is a natural antimicrobial which is limited by its insolubility and low absorption. Chitosan nanoparticles consider promising bioactive polymers in nanomedicine regarding to their antibacterial activity, non- toxic and non- immunogenic properties which also make them ideal drug delivery agents. The present study describes the synthesis of chitosan nanoparticles and chitosan-propolis nanocomposite by a facile chemical method. The physicochemical properties of chitosan nanoparticle and nanocomposite have been characterized by FTIR fingerprint spectroscopy, zeta potential and TEM microscopies. With an assessment to the application of chitosan nanoparticles and nanocomposite on Vero cell line, the safety of these particles was determined. Characterization of chitosan nanoparticle and chitosan-propolis nanocomposite indicated the spherical phase with size 26.13 nm and 30.45 nm, respectively; for the FT-IR spectroscopic analysis; nanocomposite showed the interaction between chitosan chains molecule and propolis when compared with chitosan only, indicating the actual hydrogen bonding in many sites. The zeta potential of chitosan nanoparticle and chitosan-propolis nanocomposite was 51.0± 5.92 mV and 41.0±7.55 mV, respectively. The results decisively improved the antibacterial activity of chitosan-propolis nanocomposite from minimum inhibitory concentration (0.5 μg/ml against S. aureus, 2 μg/ml against E-coli and 4 μg/ml against Salmonella).
Keywords | Chitosan, Propolis, nanocomposite, Vero cell line, Antibacterial
Received | September 19, 2019; Accepted | October 26, 2019; Published | December 12, 2019
*Correspondence | Sawsan M.A. El-Sheikh, Pharmacology Department, Faculty of Veterinary Medicine, Zagazig University, 44511, Egypt; Email: [email protected]
Citation | El-Sheikh SMA, El-Alim AAFA, Ibrahim HAF, Mobarez EA, El-Masry DMA, El-Sayed WA (2019). Preparation, characterization and antibacterial activity of chitosan nanoparticle and chitosan-propolis nanocomposite. Adv. Anim. Vet. Sci. 7(s2): 183-190.
DOI | http://dx.doi.org/10.17582/journal.aavs/2019/7.s2.183.190
ISSN (Online) | 2307-8316; ISSN (Print) | 2309-3331
Copyright © 2019 El-Sheikh et al. This is an open access article distributed under the Creative Commons Attribution License, which permits unrestricted use, distribution, and reproduction in any medium, provided the original work is properly cited.
Introduction
Propolis is a waxy, brownish and sticky substance collected by bees from buds of certain trees and plants exudates mixed with pollen and enzymes secreted by bees in order to cement or sealing cracks of their hives, protecting their hives from microbial infection by bacteria and fungi and protection against insects (Yen et al., 2017).
Depending up on the bee species, source of plant, geographical factors, collecting season and selective behavior of bees, the chemical composition and quality of propolis is defined (Isidorov et al., 2014).
Functional properties of propolis are different relating to the diversity of its chemical characterization (Ristivojevic et al., 2015; Guzelmeric et al., 2018). Propolis have presented diverse pharmacological activities as antimicrobial, anticancer, anti-inflammatory, antioxidant, antidiabetic, antiulcer and antihepatotoxic (De Groot, 2013; Huang et al., 2014; Bueno-Silva et al., 2016).
The evolution of microbial resistance and antibiotic residue due to over and misuse of antibiotics need to overcome through formulation novel natural-based antimicrobial agents (Raffi et al., 2010; Usman et al., 2013). It has been reported the difficulty in building tolerance for propolis by bacteria as a result of complexity and synergism of its constituents. Bayram and Gerçek (2017) support the antimicrobial influence of propolis against gram-negative, gram-positive bacteria and yeast like fungi considering it as alternative medicine.
Chitosan is a natural cationic polysaccharide obtained from chitin, the major component of exoskeletons of crustaceans and insects, through alkaline deacetylation process (Younes and Rinaudo, 2015; Vilar et al., 2016). Chitosan has been implemented in various pharmaceutical and biomedical applications for its unique properties such as biocompatibility, adhesiveness, biodegradability, non-toxic and non-immunogenic. Chitosan has an in vivo and in vitro applications against bacterial infections (Cheung et al., 2015), in order to the positively charged chitosan can efficiently interact with negative charge surface of bacterial cell wall causing bacterial disruption and changing the membrane permeability then DNA attachment occurred resulting in inhibition of DNA replication and finally bacterial cell death (Nagy et al., 2011).
Nanotechnology has opened up a new era in biomedical and pharmaceutical applications regarding to better bioavailability, improved therapeutic efficacy and enhanced penetrative capacity (Ong et al., 2017). Chitosan nanoparticles has received attention as a good drug delivery system regarding to its ideal physicochemical properties such as particle size, controlled drug release properties, polydispersity index, encapsulation efficiency and zeta potential and this is the main base in choice of chitosan-propolis nano-formulation (Yuan et al., 2010; Ong et al., 2017). Nanopropolis has found to be more effective as antimicrobial than propolis (Afrouzan et al., 2012). Also, Patel and Agrawal (2011), Yien et al., (2012) found that chitosan nanoparticles had more superior bioactivities than its parent chitosan.
Cytotoxicity related with nanotechnology elevated certain interest of the unique physicochemical properties (charge, size, outer coating bioactivity and concentration) and environmental conditions (photolytic, oxidative, and mechanical stability). For example, some nanoparticles were found to be cytotoxic only after oxidative and/or photolytic degradation of their core coatings. Few in vivo and in vitro studies have reported that nanoparticles could affect viability and cell growth in a dose-dependent sort (Sherif, 2016).
Salmonella usually is one of the main zoonotic pathogen included in food borne epidemics in all areas of the world and S. typhimurium as one of the most recurrent and virulent serovars producing food borne disease in animals and human (Herrero-Fresno and Olsen, 2018).
Material and Methods
Materials
- 1. Chitosan 93% degree of deacetylation in powder form, oxford Lab.,
- 2. Sodium tripolyphosphate (TPP) 99.5% was obtained from El-Gomhoria for chemicals Co.,
- 3. Propolis, glacial acetic acid was purchased from Sigma Aldrich.
- 4. Tween 80 was purchased from MP biomedical.
- 5. Vero cell line was prepared in Reference Lab for Quality control and Poultry Production (RLQP), Dokki.
- 6. Escherichia coli, Staphylococcus aureus and Salmonella typhimurium obtained from Animal Health Research Institute (AHRI), Dokki.
Methods
Chitosan nanoparticles preparation
Nanoparticles were produced based on ionic gelation of TPP (sodium tripolyphosphate) and chitosan (Calvo et al., 1997). Nanoparticles were obtained upon the addition of 4% chitosan acidic solutions (0.5% acetic acid) respectively, to solutions of TPP aqueous basic solution (0.7mg /ml); under magnetic stirring the ratio of TPP to chitosan was 1:3 at 25°C for 1hr.
Preparation of chitosan-propolis nanocomposite: according to Ong et al., (2017)
Propolis (1.6 mg/ml) was put to chitosan solution (0.5% w/v) containing Tween 80 (0.4% w/v) with constant stirring to produce chitosan-propolis nanoparticles.
Then the mixture was sonicated for 5 minutes and the TPP solution was dropped under constant stirring. The ratio 2:1 of chitosan: TPP solution should be maintained throughout the experiment.
The ultracentrifugation of the obtained supernatant at 25000 rpm for 20 minutes must be adjusted for sedimentation of the chitosan-propolis conjugated nanoparticles and then characterized the nanoformulate.
Characterization of chitosan nanoparticle and chitosan-propolis nanocomposite
It was done through Fourier transmittance Infrared FT/IR-6100 Spectrometer, High-resolution transmission electron microscopy (HRTEM) imaging JEM 2100F transmission electron microscope with accelerating voltage 200 kV and Zeta sizer Nano ZS instrument (Malvern Instruments, Worcestershire, UK).
Cytotoxicity of chitosan nanoparticle and chitosan-propolis nanocomposite
It was done through using Vero cells (African green monkey kidney cells). Vero cell lines were grown in minimal essential medium (MEM) with Eagle’s salts containing 10% fetal bovine serum, 100 IU of Benzyl penicillin B /ml, 100 μg of streptomycin/ml, amphotericin-B 100μg/ml, 2 mM L-glutamine-ml and 150 μg of G418/ml. These cells were incubated in 5% CO2 –balanced air at 37°C.
Minimun inhibitory concentration (MIC) was determined according to EUCAST (2000)
Bacterial suspensions for Escherichia coli, Staphylococcus aureus and Salmonella typhimurium were adjusted to the logarithmic-phase growth to match the turbidity of a 0.5 McFarland standard, producing approximately 108 CFU/ml. The bacteria of the same amounts were added to all tubes which then were incubated at 37 °C for 24 h. The bacteria growth was examined and matched to the control. Bacterial inoculum was prepared by suspension of freshly grown bacteria in sterile saline and was compared to a 0.5 McFarland standard.
Results
Particle size, morphology and size Distribution
The nanoparticles size and morphology are mainly determined by HRTEM which chitosan nanoparticles size had 26.15 nm narrow size distribution (polydispersity index (PdI): 0.841±3.922) which indicated that greater homogeneity could be realized. The chitosan nanoparticles are nanosphere shape with no aggregation while the chitosan-propolis nanocomposite showed nanosphere shape, no aggregation and size 29.41 nm with polydispersity index (PdI): 0.691±17.35 (Figures 1 and 2) respectively.
Chemical interaction
(Figure 3) Fourier Transform Infrared Spectroscopy analysis is distinctive molecular fingerprint and detection of functional groups in compound comparison with pure compounds.
FTIR spectra of chitosan have three peaks of characterization (3.432 cm−1 of ν (OH), 1,080 cm − 1 of ν (C O C) and 1.647 cm−1 of ν (NH) yielded in the spectrum of purified chitosan.
The propolis extract appeared typical hydrogen-bonded O–H stretch 3336 cm−1 (phenolic hydroxyl group), C=C stretches of aromatic ring at 1617, 1496 and 1450 cm−1 and flavonoids (aromatic ether C–O bond) at 1045 cm−1 as well as aromatic C–H at 877 cm−1 resulting to the angular deformation.
FT-IR spectra of propolis-chitosan nanocomposite explained the interaction between chitosan chains molecular and propolis compared with propolis and chitosan. A broad peak between 3470 and 3280 cm−1O H Interrelated N H bonds in chitosan matrix. 1580 cm−1(–C=O), 1436 cm− (NH3) protonated amine group, 1321 cm−1(OH), 1155 (CO) of the ring C–O–H, C–O–C and CH2CO930, 653, 623 cm− 1 distinguish for propolis.

Figure 1: HRTEM of chitosan nanoparticle showed nanosphere shape, no aggregation and size 26.15nm with Mag. 8000× to 16800× and 30000× to 63000× (Central lab. in NRC).
Zeta potential
Detection by using dynamic light scattering (DLS).The zeta potential is an indicator to stable and unstable suspensions. It is generally taken at either +30 or −30 millivolt (mV). The zeta potential results for the present study indicated that chitosan nanoparticle had a 51.0± 5.92 mV of concentration 0.5% acetic acid while, propolis-chitosan nanocomposite had 41.0±7.55 mV measured at pH 5 (Figure 4).

Figure 2: HRTEM of chitosan-propolis nanocomposite showed nanosphere shape, no aggregation and size 29.41 nm with Mag. 30000× to 63000× and 15000× to 36600× (Central lab. in NRC).
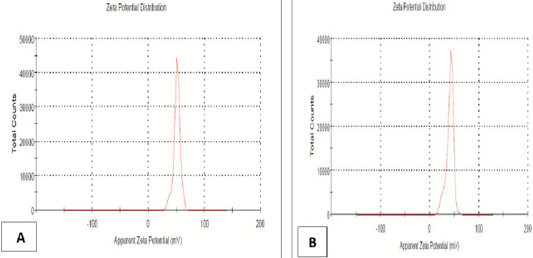
Figure 4: Zeta potential chitosan (A) and chitosan-propolis nanocomposite (B) (Central lab. in NRC).

Photo 1: Negative control Vero cells 72 hr. post inoculation (A), chitosan nanoparticle no effect to cells (B) and chitosan-propolis nanocomposite (C) after 24 hrs(1) and 72hrs(2).
Cytotoxicity
To assessment the best concentration for Nano-composite formula, different concentrations of chitosan nanoparticles and chitosan-propolis nanocomposites (1, 10, 20, 30, 25, 50, 75, 100 microliter (uM)) were inoculated on confluent sheet of Vero cells to determine the cytopathogenic effects (CPE).
The experiment of Vero cells, after 72 hours of chitosan nanoparticle inoculation showed no CPE in Vero cells as compared with control. While, the chitosan-propolis nanocomposite chosen dose was 50 mg/L (Photo 1A, B, C).
Minimum inhibitory concentration (MIC)
The chitosan-propolis nanocomposites showed bactericidal activity. The MIC values of these particles were 0.5 μg/mL, 2 μg/mL and 4 μg/mL for gram positive (S. aureus) and gram negative bacteria (E-coli and S. typhimyrium) respectively. The MIC values ofchitosan nanoparticles are 225 μg/mL for all tested microorganisms (Table 1).
Table 1: MIC of chitosan-propolis nanocomposite and chitosan nanoparticle against S. aureus, E-coli and S. typhimurium.
Bacteria | Chitosan-propolis nanocomposite |
Chitosan nanoparticle |
S. aureus | 0.5 μg/ml | 225 μg/ml |
E-coli | 2 μg/ml | 225 μg/ml |
S. typhimurium | 4 μg/ml | 225 μg/ml |
Discussion
In our present study, we successfully synthesized and optimized chitosan and chitosan-propolis nanoparticles. Particle size has an important role to obtain the optimal efficacy of nanoparticles. A reduction in the particle size could enhance the efficacy, solubility and the bioavailability of poorly water soluble drugs. Regarding to the endocytosis of small size particles, the small particles have more efficient interaction than large particles with the cell membrane (Koukaras et al., 2012; Khanmohammadi et al., 2015).
Our study recorded small sized particles compared to several studies that showed different size particles such as Ong et al. (2017) who recorded different average particle size of chitosan-propolis nanoparticles from 247.1 nm to 512.3 nm in six different formulation, Xu and Du (2003) showed that chitosan nanoparticles with various formations appeared spherical shape and were 20-200 nm diameter using TEM, Wardani et al. (2018) recorded that the chitosan nanoparticles diameter were around 500 nm and Cavalu et al. (2018) found that the formation of nanoparticles was further confirmed by laser diffraction, revealing that particle size obtained from highly dispersed mixture was in the range of 50-400 nm, with large Gaussian distribution, the maximum percentage of size distribution being at around 120 nm. These changes in the nanoparticles size could be attributed to the concentration and nature of the polymer in the organic phase, the concentration and nature of the surfactants in the aqueous phase and the polarity of the solvent (Scaffazick et al., 2003).
The present study confirmed the positive zeta potential of our nanoparticles and there was no aggregation. In similar way, Ong et al. (2017) found that the zeta potential in the different formulations were between +35.5 mV to +74.1 mV and Ong et al. (2019) reported that chitosan-proprolis nanoparticle (CPNP) had a positive zeta potential of +40 mV because of the cationic properties of the chitosan. The zeta potential is an indicator to stability of the nanoparticles which prevent aggregation or precipitation of these particles by repulsion between particles (Nair et al., 2010).
Positive zeta potential nanoparticles explained the antibacterial activity of them due to surface charge neutralization between bacterial surface and antimicrobial agents (Arakha et al., 2015).
Our present study showed the interaction between chitosan molecule and propolis through FTIR spectra. Our results reinforced by Wu et al. (2005) who recorded a new sharp peak showed at 1,632 cm−1 in chitosan-TPP nanoparticles and the 1,647cm−1 peak of – NH 2 bending vibration moved to 1,519 cm−1. It could be supposed that the TPP phosphoric groups were connected with chitosan ammonium groups in nanoparticles (Xu and Du, 2003; Wu et al., 2005).
Other study reported by Annadurai (2012) showed a peak at 3400 cm-1 corresponding to O-H stretching vibration in chitosan by chitosan nanoparticle FTIR spectra.
Our present findings were clearly showed the nontoxicity of chitosan and chitosan-propolis nanoparticles on vero cell line. Our results were supported by Nivethaa et al. (2015) who proved the safety of chitosan-gold nanocomposite on VERO cells. Moreover, Mohammed et al. (2017) demonstrated that chitosan based nanoparticles showed less cytotoxicity compared to the chitosan alone explaining the reasons to the linker attached to chitosan nanoparticle or the intracellular response was different to free material than nanoparticles.
On the other side, Gao et al. (2012) stated that some nanoparticles could induce many cytotoxic effects to some cell types so, nanotoxicology researchs are needed.
Our study proved the antibacterial activity of chitosan nanoparticles and chitosan-propolis nanocomposite. Our results were reinforced by several studies such as Prasetyo et al. (2011) who stated that nanopropolis were found to have increased bacterial activity compared to the propolis extract against S. aureus, B.subtilis, Salmonella sp. and E-coli, Park et al. (2004); Divya et al. (2017); Wardani et al. (2018) proved a good demonstration of the chitosan nanoparticles ability against wide range of bacteria, Ong et al. (2017) proved that chitosan-propolis nanoformulation introduce the ability to inhibit bacterial growth of Enterococcus faecalis that is known to be resistant to most antibiotics and had ideal physicochemical parameters, Ong et al. (2019) reported that chitosan-propolis nanoformulation showed synergism with the antibiotics suggestive of effective treatment regimens of Staphylococcus epidermidis, rifampicin, ciprofloxacin, vancomycin and doxycycline. In addition to the direct inhibitory effect on the S. epidermidis survivability and Gonsales et al. (2006), Afrouzan et al. (2012) evoked that nanopropolis was more effective against Staphylococcus aureus than the tetracycline.
On the other side, Qurbatussofa (2013) stated that nanopropolis does not have antibacterial activity against E-coli using disc diffusion method and Drago et al. (2007) reported that proplis showed activity against gram positive bacteria, but showed limited activity for gram negative bacteria.
This antimicrobial property of chitosan nanoparticles was attributed to high surface area, small size, strong curvature of the surface and charge density that enable chitosan nanoparticle to intereact with the negative charge of bacterial cell surfaces leading to their death (Shi et al., 2006; Yien et al., 2012).
Ong et al. (2017) explained that propolis extracts had a negative surface charge leading to weaker interaction between the surfaces of bacteria and propolis because of the repulsive force. The changes in zeta potential in chitosan propolis nanoparticles made it to be more effective as a potential alternative antimicrobial agent.
Conclusion
The smallest size, spherical in shape and stable nano particles were obtained through the characterization of chitosan nanoparticle and nanocomposite by FTIR fingerprint spectroscopy, zeta potential and TEM microscopies. Also, safety of these particles was achieved through the culture on Vero cell line. Nanomedicine against three pathogens in veterinary medicine could be approved in vitro by MIC demonstrating the applicability of chitosan nanoparticles and chitosan-propolis nanocomposite as a promising alternative antibacterial.
Acknowledgements
A special word of thankfulness must be offered to Dr. Hend K.Sorour, Senior researcher of Bacteriology Unit, RLQP, Animal Health Research Institute, Dokki for her help, cooperation, supplying facilities required for practical work.
Authors Contribution
Sawsan M.A. El-Sheikh designs the protocol and revises the manuscript.
Abd El-Alim F. Abd El-Alim, Hosny Abd El-Fadil Ibrahim and Elham A. Mobarez revise the manuscript.
Dalia M.A. El-Masry prepares and characterizes the nanoparticles.
Walaa A. El-Sayed drafts the manuscript and collects the data.
Conflict of interest
The authors declare that they have no conflict of interest.
ReferenceS
https://doi.org/10.5530/pj.2018.1.27