Advances in Animal and Veterinary Sciences
Research Article
Advances in Animal and Veterinary Sciences 2 (4): 233 – 238Genotypic and Phenotypic Characterization of Clinical Isolates of Staphylococcus aureus for Biofilm Formation Ability
Rashmi Goyal1*, Priscilla Kerketta1, Pavan Kumar1, Mayank Rawat2, Konasagara Nagaleekar Viswas3, Rajesh Kumar Agarwal3
- Division of Veterinary Public Health, Indian Veterinary Research Institute, Izatnagar, Bareilly 243122, India
- Division of Biological Standerdization, Indian Veterinary Research Institute, Izatnagar, Bareilly 243122, India
- Division of Bacteriology and Mycology, Indian Veterinary Research Institute, Izatnagar, Bareilly 243122, India
*Corresponding author: [email protected]
ARTICLE CITATION:
Goyal R, Kerketta P, Kumar P, Rawat M, Viswas NK, Agarwal RK (2014). Genotypic and phenotypic characterization of clinical isolates of Staphylococcus aureus for biofilm formation ability. Adv. Anim. Vet. Sci. 2 (4): 233 – 238.
Received: 2014–02–18, Revised: 2014–03–02, Accepted: 2014–03–02
The electronic version of this article is the complete one and can be found online at
(
http://dx.doi.org/10.14737/journal.aavs/2014/2.4.233.238
)
which permits unrestricted use, distribution, and reproduction in any medium, provided the original work is properly cited
ABSTRACT
The objective of the study was to characterize Staphylococcus aureus (S. aureus) isolated from human and animal clinical cases for their biofilm formation ability by genotypic and phenotypic methods. A total of 130 S. aureus strains isolated from human wound (n = 20), animal wound (n = 70) and animal mastitis (n = 40) cases were subjected to screening for 3 different biofilm associated genes (bap, icaA and icaD) and for phenotypic assessment for biofilm formation using Congo red agar, modified Congo red agar and microtitre plate assay. PCR assays were standardized for the detection of bap, icaA and icaD genes. The results indicated that icaA gene was present in 51.15% of the isolates and bap gene was present in 8.46% isolates. None of the isolates were positive for icaD gene. Human isolates (65%) had higher occurrence of icaA gene in comparison to animal isolates (49.09%). Dog wound isolates had higher occurrence of bap gene. Of the 3 methods used for phenotypic expression of biofilm by S. aureus isolates modified Congo red agar method showed 86.92% isolates to be positive, whereas by Congo red agar method only 63.07% S. aureus were found to be biofilm producer. Microtitre plate assay showed 75.38% S. aureus isolates to be biofilm producers. A good correlation was observed between genotypic and phenotypic biofilm formation ability of the isolates. Bap gene contained isolates showed higher biofilm producing ability compare to icaA gene harbored isolates.
INTRODUCTION
Staphylococcus aureus is a Gram–positive bacterium and is an important human and animal pathogen. The S. aureus causes a wide variety of infections ranging from mild skin infections, to life–threatening diseases such as bacteremia. The pathogenesis of S. aureus is attributed to the combined effect of extracellular factors and toxins, together with the invasive properties of the strain such as adherence, biofilm formation, and resistance to phagocytosis. There is general agreement that biofilms are the basis for persistent or chronic bacterial infections (Costerton et al., 1999). The implication of biofilms in chronic infections has triggered an increasing interest in the organization of genes involved in biofilm formation (Caizza and O’Toole, 2005; Tormo et al., 2005). The icaADBC cluster, an operon present in S. aureus and S. epidermidis, participates in biofilm formation by encoding proteins involved in the synthesis of a biofilm matrix polysaccharide (Cucarella et al., 2004). The ica operon was first identified and studied most extensively in S. epidermidis and was later shown to be present in S. aureus (Gotz, 2002). Most S. aureus strains appear to contain the entire ica operon, although there are reports to the contrary.
IcaA and icaD genes have been reported to play a significant role in biofilm formation in S. aureus and S. epidermidis (Gotz, 2002). The ica locus has been detected in majority of the mastitic S. aureus isolates indicating its potential role as a virulence factor in the pathogenesis of mastitis in ruminants (Ciftci et al., 2009, Milanov et al., 2010). Little information is available regarding genotypic characterization of S. aureus of animal and human clinical origin with reference to intercellular adhesion genes and its association with phenotypic characters of Indian isolates (Vasudevan et al., 2003, Dhanawade et al., 2010).
More recently, Cucarella et al., (2001) identified a surface protein (Bap, Biofilm Associated Protein) implicated in S. aureus biofilm formation. Vautor et al., (2008) has reported that the bap protein is a member of proteins playing a role in biofilm formation in many bacteria and they share common structural features as they have a high molecular weight and contain a core domain of tandem repeats. Bap gene has been identified in a small proportion of S. aureus from bovine mastitis (Cucarella et al., 2001). Many studies have yielded negative results for the presence of bap gene in human and animal cases (Arciola et al., 2001; Vasudevan et al., 2003; Vancraeynest et al., 2004; Nitzsche et al., 2007, Vautor et al., 2008) indicating low prevalence of this gene. No information is available about Indian S. aureus isolates.
A number of methods have been developed for cultivation and quantification of biofilm, such as tube test, microtiter plate test, radiolabeling, microscopy, Congo red agar plate test, etc. (Deighton et al., 2001; Mathur et al., 2006; Agarwal et al., 2011). Nevertheless, the microtiter plate and Congo red agar method remains among the most frequently used assays for investigation of biofilm (Vasudevan et al., 2003; Knobloch et al., 2002).
Therefore, the present study was undertaken to characterize Staphylococcus aureus isolated from human and animal clinical cases for their biofilm formation ability by genotypic and phenotypic methods.
MATERIALS AND METHODS
Bacterial strains
The bacterial stains included in the study are listed in the table 1. Most of the strains (III) were isolated in an earlier study in our laboratory in the year 2013. Twenty–nine bovine mastitis strains isolated in the year 2005 were procured from the repository of the Division of Standardization, Indian Veterinary Research Institute, Izatnagar, India. All the strains were tested for their purity, morphological and biochemical characteristics and were maintained by periodical sub culturing in brain heart infusion (BHI) broth.
Primers used in the study for detection of icaA, icaD and bap genes are listed in table 2.
PCR Protocol
All the 130 S. aureus isolates were subjected to amplification of icaA icaD and bap genes. The reaction mix invariably consisted of 5 µl of bacterial DNA, 2.5 µl of 10x PCR buffer for Taq polymerase [100 mM Tris HCl, pH 8.3, 500 mM KCl, 15 mM MgCl2, 0.01% gelatin], 1.5 µl of 2.5 mM dNTP, 10 pmol of each forward and reverse primers, and 1 U of Taq polymerase. The final volume of 25 µl was made up by using milli Q water. For icaA and bap genes an annealing temperature of 55ºC was used, whereas for the amplification of icaD gene annealing was done at 49ºC. The cycling conditions used were an initial denaturation of 5 min at 94ºC, 35 cycle of 45 sec denaturation at 94ºC, 45 sec annealing at optimum temperature and 45 sec extension at 72ºC. Appropriate positive and negative controls were added in each of the PCR run. The PCR products were confirmed by agarose gel (1%) electrophoresis.
Phenotypic Expression of Biofilm
Biofilm formation ability of all the S. aureus isolates was studied by microtitre plate method and Congo red binding assay and modified Congo red assay.
Microtitre Plate Assay
The test was performed using tryptone soy broth containing 1% glucose (TSB + 1% Glucose). The isolates were first inoculated in the TSB + 1% glucose and incubated for 24 h at 37ºC. From each individual culture, 20 µl of TSB+1% glucose broth were dispended in the wells of sterile 96–well flat bottomed microtitre plate (Greiner bio–one) and kept for incubation at 37ºC for 48 h under aerobic conditions. Each strain was inoculated into at least 8 wells. The control well contained only TSB+1% glucose without inoculation. After incubation of plates, unbound cells were removed by inversion of microtitre plate, followed by vigorous tapping on absorbent paper. After that, adhered cells were fixed at 80ºC for 30 min.
Adhered cells on the bottom and side of the wells were stained by addition of 220 µl of crystal violet (0.5%) for 15 min and excess stain was rinsed off. The stain was removed by exhausting washing with distilled water. The plates were then allowed to air dry. After drying, 220 µl of decolouring solution (ethanol 80% and acetone 20%) was added to each well for 15 min. The absorption of eluted stain was measured at 590 nm in ELISA reader. Data obtained from above experiments was subjected to statistical analysis as per standard procedure of Snedecor and Cochran (1989).
Detection of Biofilm Associated Genes by PCR
Primers
Congo red Agar Assay
For Congo red assay two different methods were used to prepare plates. In first, Congo agar plates were prepared by adding brain heart infusion broth– 52 g, sucrose– 36 g, Congo red dye– 0.8 g and 2% agar. Modified Congo red agar was performed as suggested by Mariana et al. (2009). Isolates producing weak black, black or very black colonies were considered as biofilm producer and isolates with red colonies were considered as non biofilm producers.
RESULTS
Occurrence of Biofilm Forming Genes among S. aureus Isolates by PCR
The biofilm forming ability of the S. aureus isolates was assessed by studying occurrence of 3 different biofilm associated genes viz., icaA, icaD and bap. PCR assay was standardized for all the 3 genes by empirical variation of annealing temperature (50º–60ºC), concentration of primers (10 pmol to 15 pmol), template volume (3µl to 5µl) and the cycling conditions. PCR under optimized conditions yielded the desired sized product.
A comparison of occurrence of the 3 biofilm associated genes among all the S. aureus isolates indicated that icaA gene was present in 51.15% of isolates, followed by bap 8.46% genes (Table 3, Figure 1 and 2). None of the isolates were positive for icaD gene. Human isolates (65%) had more occurrence of icaA gene in comparison to animal isolates (49.09%). However, none of the human isolates carried bap gene. Among different animal isolates, dog wound isolates had higher occurrence of the bap gene.
Phenotypic Characterization of Biofilm Ability of S. aureus
Biofilm production ability was assessed by microtitre plate assay (Table 4). Out of 130 isolates, 2 isolates (1 human, 1 mastitis) produced very strong biofilm, 11 isolates (1 cattle wound, 2 cattle mastitis, 7 dog, 1 human, 1 mastitis isolates) produced strong biofilm, 32 isolates (3 cattle wound, 11 dog, 1 human and 17 mastitis isolates) produced moderate biofilm, 53 isolates (12 cattle wound, 10 cattle mastitis, 17 dog, 1 each of horse and goat, 11 human) produced weak biofilm. Remaining 32 isolates did not produce any biofilm. Overall, 75.38% isolates were adjusted as biofilm producers.
Biofilm production ability was also assessed by Congo red method (Table 5). Out of 130 isolates, 4 isolates (2 human and 2 mastitis isolates) produced very black colonies, 19 isolates (2 human, 2 cattle wound, 5 mastitis, 2 goat and 8 dog wound isolates) produced black colonies, and 59 (6 human, 23 dog, 6 cattle wound, 22 cattle mastitis, 1 goat and 1 horse wound) produced weak black colonies. Remaining 48 isolates produced red colonies, indicating them to be non biofilm producers.
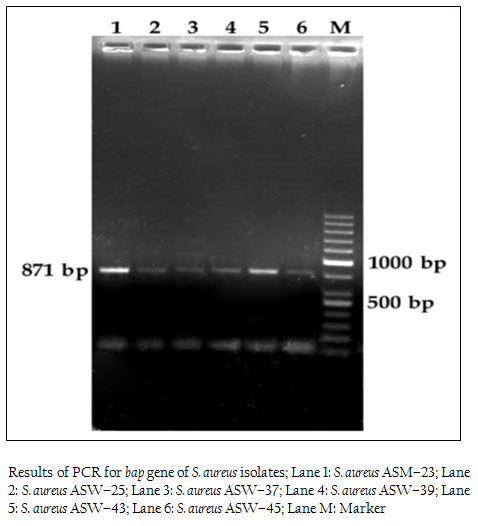
Figure 1: Results of PCR for bap gene of S. aureus isolates; Lane 1: S. aureus ASM–23; Lane 2: S. aureus ASW–25; Lane 3: S. aureus ASW–37; Lane 4: S. aureus ASW–39; Lane 5: S. aureus ASW–43; Lane 6: S. aureus ASW–45; Lane M: Marker
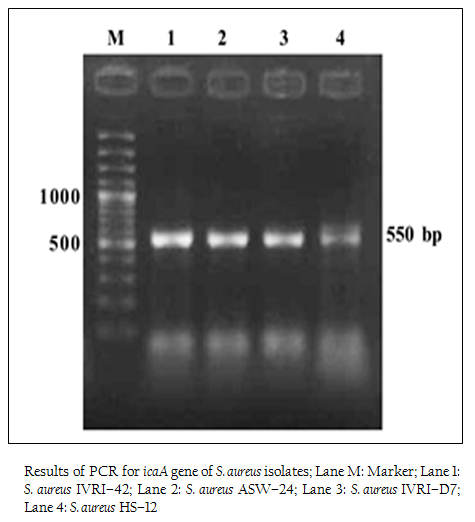
Figure 2: Results of PCR for icaA gene of S. aureus isolates; Lane M: Marker; Lane 1: S. aureus IVRI–42; Lane 2: S. aureus ASW–24; Lane 3: S. aureus IVRI–D7; Lane 4: S. aureus HS–12
On modified Congo red agar, out of 130 isolates, 5 isolates produced very black colonies, 26 isolates produced black colonies, 82 isolates produced weak black colonies and 17 isolates showed red colonies (Table 6).
Comparison of genotypic and phenotypic biofilm characteristics of the isolates revealed that the all the 11 phenotypically negative isolates were also negative for icaA and bap genes (Table 7). Of the 119 phenotypically positive isolates 56.30% isolates demonstrated the presence of icaA gene, whereas 9.24% isolates were positive for bap gene
DISCUSSION
The S. aureus has been shown to posses the capability to form biofilm (Costerton et al., 1999; Branda et al., 2005; Heilmann et al., 1997; Caizza and O’Toole, 2005; Tormo et al., 2005; Cucarella et al., 2001; Vasudevan et al., 2003, Dhanawade et al., 2010; Vautor et al., 2008), which help bacteria to adhare to an inert or living surface (Costerton et al., 1999) and is able to adhere and form biofilm consequently causing severe morbidity and infection (Sheagren, 1984; Waldvogel et al., 1995). The ability to produce biofilm is also the most important reason for eradication of infection and recurrent infections of mammary glands in bovine mastitis caused by S. aureus (Melchior et al., 2006b). Biofilm production enables adhesion of bacteria to the epithelium of mammary glands. It also facilitates persistence of micro–organisms in the host tissue by protecting the bacterial cells against the mechanisms of the host defense (Melchior et al., 2006a, 2007). Production of biofilm requires the presence of the intracellular adhesion locus gene cluster icaADBC (Cramton et al., 1999) and strains harboring the icaADBC cluster are potential biofilm producers. In addition, biofilm– associated protein (Bap) is also for the primary attachment and cells’ accumulation (Cucarella et al., 2001; Lasa and Penadés, 2006).
In our study, we examined the ability of biofilm production in S. aureus by detecting icaA and icaD genes in all isolates. We tested a total of 130 isolates for the presence of icaA and icaD genes. Of the 110 animal isolates 54 (49.09%) isolates were positive for icaA gene, but no isolate was positive for icaD gene. The distribution of icaA gene among different animal isolates was 44.4% (8/18) in cattle wound, 65% (26/40) in cattle mastitis, 37.5% (18/48) in dog wound, 100% (1/1) in horse wound, 33% (1/3) in goat wound. Earlier workers have reported variation in the occurrence of these genes. Melchior et al. (2009) found 74 out of 99 strains isolated from mastitis in Netherlands to be positive. Cifti and coworkers examined the group of 59 isolates from mastitis and found only 16 icaA positive stains, 38 strains harboured the icaD gene and 15 of them contained both genes (Cifti et al., 2009). Among the group of 102 S. aureus mastitis isolates from India, only 36 revealed the presence of both genes (Dhanawade et al., 2010). On the other hand, several authors showed presence of the ica locus genes in all S. aureus clinical isolates analyzed in their studies (Fowler et al., 2001; Rohde et al., 2001; Knobloch et al., 2002; Asthan and Shamsudin, 2011, Szweda et al., 2012). These variations could be due to circulations of different clones of S. aureus in different regions.
In the case of strains isolated from human wound infection, 13 isolates out of 20 were icaA positive. But none of the isolates possessed icaD gene. In contrast, 36/46 isolates from auricular infections in Tunisie were icaA and icaD positive (Zmantar et al., 2010), while Grinholc and Coworkers were not able to detect the presence of icaD gene in case of 27 strains among the tested group of 302 clinical MRSA isolates, whereas all of them harbored the icaA gene (Grinholc et al., 2007). Rohde et al. (2007) found that all S. aureus had the icaA gene. On the other hand, Arciola et al. (2001) suggested that 60.86% strains of S. aureus had icaA and icaD. It has been demonstrated that most of the S. aureus strains contain the entire ica operon (Cramton et al., 1999; Arciola et al., 2001a).
We also studied the occurrence of bap gene among the Staph aureus isolates. PCR assay was successfully standardized employing self–designed primers. Eleven (8.46%) isolates out of 130 showed the presence of bap gene, of which 2 were from cattle wound, 1 from cattle mastitis and 8 from dog wound samples. Bap gene was not present in any of the goat, horse and human isolates. These results are in agreement with the previous reports on S. aureus by Cucarella et al. (2001) who found only 5% isolates positive for bap gene. Szweda et al. (2012) did not report bap gene in any of isolates in his study in Poland. Similar, results were found in S. aureus isolates of different origin, such as human, sheep, goat, bovine, pig, poultry, horse and rabbit (Vancraeynest et al., 2004; Nitzsche et al., 2007; Vautor et al., 2008; Melchior et al., 2009), Vantor and cowoekers (2008), hypothesized that the bap gene has not spread among the S. aureus isolates of animal and human origin and its prevalence is very low.
Interestingly, in contrast bapA gene has shown to be high conserved in Salmonella (Lasata et al., 2006; Lassa and Penades, 2006). But in S. aureus, only some isolates (5%) from clinical mastitis cases and other clinical cases in animal and human are reported to carry bap gene (Cucarella et al., 2001). The low prevalence of bap gene in S. aureus may be because of either the gene is recently acquired in the pathogenecity island SaPIbov2, a mobile genetic element or the horizontal transfer is not easy (Vautor et al., 2008).
Phenotypic expression of biofilm formation was studied by Congo red agar assay, modified Congo red assay and microtiter method. In present study, out of 130 strains of S. aureus isolates from animal and human clinical cases, 63.07% strains were considered biofilm producer by Congo red agar method. In which, 3.07% isolates produced very black colonies, 14.61% produced black colonies, 45.38% produced weak black colonies and remaining 63.07% produced red colonies. Previous work on biofilm formation carried out by Krukowski et al. (2008), reported 42% biofilm producing S. aureus by CRA method. In another study, 42% isolates were found to be biofilm producer (Dziekiewicz – Mrugasiewicz et al., 2008). Szweda et al. (2012) found 57% positive isolates by CRA method.
Modified Congo red method was also used to study the biofilm production ability of S. aureus (Mariana et al., 2009). In this study, we found 86.92% isolates to be positive for biofilm production. Similar findings have been reported by Atshan et al. (2012). The microtiter plate method remains among the most frequently used assays for investigation of biofilm. In this study, we also optimized microtitre plate method and used it for testing all the 130 Staph aureus isolates for biofilm formation ability. Of these 130 isolates, 75.38% were found to be slime producers by microtitre plate assay. Gundogan et al. (2006) found that 58 out of 110 S. aureus strains were slime producers by this method. Furthermore, Vasudevan et al. (2003) demonstrated that 32 of 35 S. aureus isolates were slime positive and Zmantar et al. (2010) found that 26 out of 46 strains of S. aureus (56.5%) were slime producers. However, Atshan et al. (2012) found 100% isolates to be biofilm producers.
Comparison of genotypic and phenotypic biofilm characteristics of the isolates revealed that absence of either of 2 genes studied (icaA and bap) clearly indicated that the S, aureus isolates were negative for biofilm production. Occurrence of these 2 genes was variable among the phenotypically positive S. aureus isolates, indicating that there are other factors involved in biofilm formation. However, the bap gene containing isolates showed higher biofilm producing ability in both, microtitre plate and Congo red assays (result not shown). We conclude that the presence of bap gene in S. aureus isolate will definitely indicate high biofilm production ability.
ACKNOWLEDGEMENTS
Authors are thankful to Director, Indian Veterinary Research Institute, Izatnagar for providing necessary facilities for research work.
REFERENCES
Agarwal RK, Singh S, Bhilegaonkar KN, Singh VP (2011). Optimization of microtitre plate assay for the testing of biofilm formation ability in different Salmonella serotypes. Int. Food Res. J. 18: 1493 – 1498.
Arciola CR, Baldassarri L, Montanaro L (2001). Presence of icaA and icaD genes and slime production in a collection of Staphylococcal strains from catheter–associated infections. J. Clin. Microbiol. 39: 2151 – 2156.
http://dx.doi.org/10.1128/JCM.39.6.2151-2156.2001
PMid:11376050 PMCid:PMC88104
Atshan SS, Shamsudin MN (2011). Evaluation of phenotypic and genotypic detection methods for biofilm–forming methicillin sensitive and methicillin–resistant Staphylococcus aureus clinical isolates. Ann. Microbiol. DOI 10.1007/s13213 – 011 – 0201 – 1.
Atshan SS, Shamsudin MN, Sekawi Z, Lung,LTT, Hamat RA, Karunanidhi A, Ali AM, Ghanaznavi–Rad E, Ghasemzadeh–Moghaddam H, Sen JSC, Nathan JJ, Pei CP (2012). Prevalence of adhesion and regulation of biofilm– related genes in different clones of Staphylococcus aureus. J. Biomed. Biotechnol.doi : 10.1155/2012/976972.
http://dx.doi.org/10.1155/2012/976972
Branda SS, Vik A, Friedman L, Kolter R (2005). Biofilm: the matrix revisited. Trends Microbiol. 13: 20 – 26.
http://dx.doi.org/10.1016/j.tim.2004.11.006
PMid:15639628
Caiazza NC, O'Toole GA (2005). SadB is required for the transition from reversible to irreversible attachment during biofilm formation by Pseudomonas aeruginosa PA14. J. Bacteriol. 186: 4476 – 4485.
http://dx.doi.org/10.1128/JB.186.14.4476-4485.2004
PMid:15231779 PMCid:PMC438627
Ciftci A, Findik A, Onuk EE, Savasan S (2009). Detection of methicillin resistance and slime factor production of Staphylococcus aureus in bovine mastitis. Brazil . J. Microbiol. 40: 254 – 261.
Costerton JW, Stewart PS, Greenberg EP (1999). Bacterial biofilms: a common cause of persistent infections, Science. 284 (5418): 1318 – 1322.
http://dx.doi.org/10.1126/science.284.5418.1318
PMid:10334980
Cramton SE, Gerke C, Schnell NF, Nicholsw W, Götz F (1999). The intercellular adhesion (ica) locus is present in Staphylococcus aureus and is required for biofilm formation. Infect. Immun. 67: 5427 – 5433.
PMid:10496925 PMCid:PMC96900
Cucarella C, Solano C, Valle J, Amorena B, Lasa I, Penades JR (2001). Bap, a Staphylococcus aureus surface protein involved in biofilm formation. J. Bacteriol. 183: 2888 – 2896.
http://dx.doi.org/10.1128/JB.183.9.2888-2896.2001
PMid:11292810 PMCid:PMC99507
Cucarella C, Tormo MA, U’beda C, Trotonda MP, Monzon M, Peris C, Amorena B, Lasa I, Penades JR (2004). Role of biofilm–associated protein bap in the pathogenesis of bovine Staphylococcus aureus. Infect. Immun. 72: 2177 – 2185.
http://dx.doi.org/10.1128/IAI.72.4.2177-2185.2004
PMid:15039341 PMCid:PMC375157
Deighton MA, Capstick J, Domalewski E, VanNguyen T (2001). Methods for studying biofilms produced by Staphylococcus epidermidis. Meth. Enzymol. 336 :177 – 195.
http://dx.doi.org/10.1016/S0076-6879(01)36589-8
Dhanawade NB, Kalorey DR, Srinivasan R, Barbuddhe SB, Kurkure NV (2010). Detection of intercellular adhesion genes and biofilm production in Staphylococcus aureus isolated from bovine subclinical mastitis. Vet. Res. Commun. 34: 81 – 89.
http://dx.doi.org/10.1007/s11259-009-9326-0
PMid:19902374
Dziekiewicz–Mrugasiewicz M, Rzewuska M, Stefanska J, Jakubczak A (2008). Select pathogenic characteristics as well as the genotypic diversity of Staphylococcus aureus strains isolated from milk of cows in the north–east region of Poland. Medycyna. Wet. 64: 961 – 1072.
Fowler VG, Fey PD, Reller LB, Chamis AL, Corey GR, Rupp ME (2001). The intercellular adhesion (ica) locus is present in clinical isolates of Staphylococcus aureus from bacteremic patients with infected and uninfected prosthetic joints. Med. Microbiol. Immunol. 189: 127 – 131.
http://dx.doi.org/10.1007/s430-001-8018-5
Gotz F (2002). Staphylococcus and biofilms. Mol. Microbiol. 43: 1367 – 1378.
http://dx.doi.org/10.1046/j.1365-2958.2002.02827.x
PMid:11952892
Grinholc M, Wegrzyn G, Kurlenda J (2007). Evaluation of biofilm production and prevalence of the icaD gene in methicillin–resistant and methicillin–susceptible Staphylococcus aureus strains isolated from patients with nosocomial infections and carriers. FEMS Immunol. Med. Microbiol. 50: 375 – 379.
http://dx.doi.org/10.1111/j.1574-695X.2007.00262.x
PMid:17537178
Gundogan N, Citak S, Turan E (2006). Slime production, DNase activity and antibiotic resistance of Staphylococcus aureus isolated from raw milk, pasteurised milk and ice cream samples. Food Cont. 17: 389 – 392.
http://dx.doi.org/10.1016/j.foodcont.2005.01.006
Heilmann C, Hussain M, Peters G, Gotz F (1997). Evidence for autolysin–mediated primary attachment of Staphylococccus epidermidis to a polystyrene surface. Mol. Microbial. 24: 1083 – 1091.
http://dx.doi.org/10.1046/j.1365-2958.1997.4101774.x
Knobloch JK, Horstkotte MA, Rohde H, Mack D (2002). Evaluation of different detection methods of biofilm formation in Staphylococcus aureus. Med. Microbiol. Immunol. 191: 101 – 106.
http://dx.doi.org/10.1007/s00430-002-0124-3
PMid:12410349
Krukowski H, Szymankiewicz M, Lisowski A (2008). Slime production by Staphylococcus aureusstrains isolated from cases of bovine mastitis. Polish J. Microbiol. 57: 253 – 255.
PMid:19004247
Lasa I, Penades JR (2006). Bap: a family of surface proteins involved in biofilm formation. Res. Microbiol. 157: 99 – 107.
http://dx.doi.org/10.1016/j.resmic.2005.11.003
PMid:16427771
Latasa C, Solano C, Penades JR, Lasa I (2006). Biofilm– associated proteins. C. R. Biol. 329: 849 – 857.
http://dx.doi.org/10.1016/j.crvi.2006.07.008
PMid:17067927
Mariana NS, Salman SA, Neela V, Zamberi S (2009). Evaluation of modified Congo red agar for detection of biofilm produced by clinical isolates of methicillin resistance Staphylococcus aureus. African J. Microbiol. Res. 3(6): 330 – 338.
Melchior MB, Vaarkamp H, Fink–Gremmels J (2006b). Bio–films: A role in recurrent mastitis infections. Vet. J. 171: 398 – 407.
http://dx.doi.org/10.1016/j.tvjl.2005.01.006
PMid:16624706
Melchior MB, Fink–Gremmels J, Gaastra W (2007). Extended antimicrobial susceptibility assay for Staphylococcus aureus isolates from bovine mastitis growing in biofilm. Vet. Microbiol. 125: 141 – 149.
http://dx.doi.org/10.1016/j.vetmic.2007.05.019
PMid:17600640
Melchior MB, Van Osch MHJ, Graat RM, Duijkeren EV, Mevius DJ, Nielen M, Gaastra W, Fink–Gremmels J (2009). Biofilm formation and genotyping of Staphylococcus aureus bovine mastitis isolates: Evidence for lack of penicillin–resistance in Agr–type II strains. Vet. Microbiol. 137: 83 – 89.
http://dx.doi.org/10.1016/j.vetmic.2008.12.004
PMid:19150182
Milanov D, Lazic S, Vidic B, Petrovic J, Bugarski D, Seguljev Z (2010). Slime production and biofilm forming ability by Staphylococcus aureus bovine mastitis isolates. Acta. Vet–Beorgad. 60: 217 – 226.
Nitzsche S, Zweifel C, Stephan R (2007). Phenotypic and genotypic traits of Staphylococcus aureus strains isolated from pig carcasses. Vet. Microbiol. 120: 292 – 299.
http://dx.doi.org/10.1016/j.vetmic.2006.10.027
PMid:17141430
Rohde H, Burandt EC, Siemssen N (2007). Polysaccharide intercellular adhesin or protein factors in biofilm accumulation of Staphylococcus epidermidis and Staphylococcus aureus isolated from prosthetic hip and knee joint infections. Biomaterials. 28: 1711 – 1720.
http://dx.doi.org/10.1016/j.biomaterials.2006.11.046
PMid:17187854
Rohde H, Knobloch JKM, Horstkotte MA, Mack D (2001). Correlation of Staphylococcus aureus icaADBC genotype and biofilm expression phenotype. J. Clin. Microbiol. 39: 4595 – 4596.
http://dx.doi.org/10.1128/JCM.39.12.4595-4596.2001
PMid:11797608 PMCid:PMC88600
Sheagren JN (1984). Staphylococcus aureus. The persistent pathogen (second of two parts). New engl. J. Med. 310: 1368 – 1373.
http://dx.doi.org/10.1056/NEJM198405313102206
http://dx.doi.org/10.1056/NEJM198405243102107
PMid:6371530
Szweda P, Schielmann M, Milewski S, Frankowsk A, Jakubczak A (2012). Biofilm Production and presence of ica and bap genes in Staphylococcus aureus strains isolated from cows with mastitis in the Eastern Poland. Polish J. Microbiol. 61: 65 – 69.
PMid:22708349
Tormo MA, Knecht E, Götz F, Lasa I, Penadés JR (2005). Bap–dependent biofilm formation by pathogenic species of Staphylococcus: evidence of horizontal gene transfer. Vet. Microbiol. 151: 2465 – 2475.
http://dx.doi.org/10.1099/mic.0.27865-0
PMid:16000737
Vancraeynest D, Hermans K, Haesebrouck F (2004). Genotypic and phenotypic screening of high and low virulence Staphylococcus aureus isolates from rabbits for biofilm formation and MSCRAMMs. Vet. Microbiol. 103: 241 – 247.
http://dx.doi.org/10.1016/j.vetmic.2004.09.002
PMid:15504595
Vasudevan P, Nair MK., Annamalai T, Venkitanarayanan KS (2003). Phenotypic and genotypic characterization of bovine mastitis isolates of Staphylococcus aureus for biofilm formation. Vet. Microbiol. 92: 179 – 185.
http://dx.doi.org/10.1016/S0378-1135(02)00360-7
Vautor E, Abadie G, Pont A, Thiery R (2008). Evaluation of the presence of the bap gene in Staphylococcus aureus isolates recovered from human and animals species. Vet. Microbiol. 127: 407 – 411.
http://dx.doi.org/10.1016/j.vetmic.2007.08.018
PMid:17881161
Waldvogel FA (1995). In: principles and practices of infectious diseases. Mandell gl, bennett je, dolin r, ed. Wiley. New York. pp 1754 – 1777.
Zmantar T, Kouidhi B, Miladi H, Mahdouani K, Bakhrouf A (2010). A Microtiter plate assay for Staphylococcus aureus biofilm quantification at various pH levels and hydrogen peroxide supplementation. New Microbiol. 33: 137 – 145.
PMid:20518275
Zmantar T, Kouidhi B, Miladi H, Mahdouani K, Bakhrouf A (2010). A Microtiter plate assay for Staphylococcus aureus biofilm quantification at various pH levels and hydrogen peroxide supplementation. New Microbiol. 33: 137 – 145.
PMid:20518275