Advances in Animal and Veterinary Sciences
Research Article
Influence of Selenium Nanoparticles on The Effects of Poisoning with Aflatoxins
Atef A. Hassan1, Mogda K. Mansour2, Rasha M. H. Sayed-Elahl1*, Hanan A. Tag El-Din2, Mohamed E.A. Awad2, Eman M. Younis2
1Department of Mycology and Mycotoxins; 2Department of Biochemistry, Toxicology and Feed Deficiency, Animal Health Research Institute (AHRI), Agriculture Research Center (ARC), Giza, Egypt.
Abstract | The present study was undertaken for the induction of hepatotoxicity by aflatoxicosis and ameliorating their toxic effects in rats by selenium nanoparticles (Se-NPs). Biochemical parameters of all treated rats detected that aflatoxin B1 (AFB1) caused a significant decrease in total protein values and electrophoretic patterns, increase in levels of AST and ALT enzymes and decrease in urea. Aflatoxin B1 induced a marked elevation of TNF-α and IL-6 activities and decreased levels of iron, TIBC, UIBC and transferrin. The inflammation caused by AFB1 lead to elevation of hepcidin and, decrease in erythropoietin (EPO) hormones. These changes were improved by administration of Se-NPs to aflatoxicated rats and associated with successful degradation of aflatoxins residues in liver tissues. While, the administration of high dose of Se-NPs (0.5 mg/kg) to aflatoxicated rats resulted in a significant elimination of AFB1 from liver tissues than the low dose (0.3 mg/kg). Hence, it was concluded that Se-NPs can be administrated to the animal for improving the health status from adverse effects of the aflatoxicosis.
Keywords | Se-NPs, Aflatoxicosis, Interleukins, Iron parameters, Hepcidin, EPO
Received | June 01, 2020; Accepted | August 25, 2020; Published | September 20, 2020
*Correspondence | Rasha M. H. Sayed-Elahl, Department of Mycology and Mycotoxins, Animal Health Research Institute (AHRI), Agriculture Research Center (ARC), Giza, Egypt; Email: [email protected]
Citation | Hassan AA, Mansour MK, Sayed-Elahl RMH, El-Din HAT, Awad MEA, Younis EM (2020). Influence of selenium nanoparticles on the effects of poisoning with aflatoxins. Adv. Anim. Vet. Sci. 8(s2): 64-73.
DOI | http://dx.doi.org/10.17582/journal.aavs/2020/8.s2.64.73
ISSN (Online) | 2307-8316; ISSN (Print) | 2309-3331
Copyright © 2020 Hassan et al. This is an open access article distributed under the Creative Commons Attribution License, which permits unrestricted use, distribution, and reproduction in any medium, provided the original work is properly cited.
INTRODUCTION
Worldwide problems of progressive human population increase the need for hygienic food and feeds. Hence, animal health and their production gained huge attention to avoid economic losses and elevated hygienic safety of human food. Mycosis and mycotoxicosis are important causes of reduced animal production which have deleterious effects on health (FAO, 2007). Aflatoxin B1 (AFB1), being a metabolite of A. flavus and A. parasiticus, is the most significant aflatoxin regarding animal health and a common dietary contaminant particularly in hot and humid climate regions (Partanen et al., 2010).
The AFB1 toxicity related to their metabolic activity and has been recently proven to be genotoxic, cause immunosuppression, liver cirrhosis and Hepatic carcinoma (Richard, 2007; Wu et al., 2009). Other effects of aflatoxicosis include stunted growth, low feed conversions, anemia and changes in hormonal profile (Mogda et al., 2014). It can potentiate the oxidative stress on cells and cause destruction of cytoplasmic contents (Mary et al., 2012). The active intermediate, AFB1-exo-8, 9-epoxide, can bind with DNA causing its destruction (Wild and Turner, 2001) Other effects include damaging macrophages causing defects in transporting antigen-antibody-complement complexes by the macrophage system (Wang et al., 2013). Therefore, numerous detoxification strategies have been proposed to ameliorate the dangerous actions of aflatoxins by using antioxidants to prevent oxidative reactions caused by toxins (Hassan et al., 2016). Dietary selenium has several benefits for humans and animals (Surai, 2006), it helps in the regulation of thyroid hormone metabolism, cell growth and antioxidant defense systems (Pappas and Zoidis, 2012).
Recently, Se-NPs had significant beneficial actions and a nanostructure of lower toxicity than crude selenium (Zhang and Spallholz, 2011). Generally, the application of nano-minerals in several animal-health-related fields showed more significance than bulk materials regarding growth promotion, immunomodulation, antibacterial effects and enhanced livestock and poultry reproduction. (Mishra et al., 2009 and Partha et al., 2016). Therefore, the present study aimed to detect the availability of use Se-NPs to ameliorate the adverse effect of aflatoxicosis on some biochemical, immunological and hormonal parameters.
MATERIAL AND METHODS
Ethical approval
This study was approved by the Institutional Animal Health Research Ethics Committee and follows local laws and regulations.
Feed samples
One hundred and twenty samples of feed and feedstuffs were collected (yellow corn, wheat bran, hay, processed animal feeds, poultry feed and silage, 20 of each). The feeds were obtained from farms observed disease disorders in polyethylene bags and kept fridge at 5-8 oC till subjected to laboratory examination.
Experimental animals
Thirty adult male albino rats weighing 120-150 gm were used in the present study. The animals were obtained from the animal house in the Ophthalmology Research Center, Giza, Egypt. They were kept under observation for two weeks before the onset of the experiment to exclude any undercurrent infection. The animals were kept at room temperature and exposed to natural daily light-dark cycles. Rats were fed ad libitum and clean water was continuously available.
Isolation and identification of fungi
The feed specimens were prepared and examined mycological as well as the technique recommended by ISO (2008). While, the recovered fungi were identified using the method of Pitt and Hocking (2009).
production and estimation of aflatoxins on yellow corn (Smith, 1997)
The aflatoxigenic A. flavus strains that were recovered from animal feed at the Mycology laboratory were used for aflatoxin production on yellow corn. In a flask containing 100 gm of finely ground yellow corn and 40-50 ml of distilled water were mixed and autoclaved at 121 ºC for one hour. The flask was shaken to prevent cooking of yellow corn. It was inoculated with spore suspension of 2 slants of A. flavus and incubated for 4 weeks at 25-28 ºC. After the end of incubation period, the corn was removed from flasks, dried; finely ground and 50 gm of each were subjected for estimation of aflatoxins.
Detection of aflatoxins in feeds by TLC (AOAC, 2000)
Fifty grams of each feed samples were finely ground and thoroughly mixed in an electric mill, transferred to a 500 ml glass stopper Erlynmeyar flasks for the extraction and purification of aflatoxins. After purification, the extract was evaporated till dryness. The residue was cooled and stored at 0 ºC for chromatography study on thin layer plates for detection of the aflatoxin.
Synthesis and characterization of selenium nanoparticles (Verma and Maheshwari, 2018)
Se-NPs were synthesized by the chemical reduction of sodium selenite by glutathione (reduced form) and stabilized by bovine serum albumin (BSA). Specifically, 3 ml of 25 mM Na2SeO3, 3 mL of 100 mM GSH, and 0.15 g BSA were added to 9 ml of double-distilled water (dH2O) in a sterile cabinet. After mixing the reactant solution, 1M NaOH was added to bring the pH of the solution to the alkaline media. Se-NPs were formed immediately following the addition of NaOH as visualized by a color change of the reactant solutions from clear white to clear red color.
The prepared Se-NPs were subjected to further identification by UV-visible spectra of each solution were measured in a SHIMADZU UV-1800 double beam digital spectrophotometer. XRD patterns were obtained on a Philips X’pert MPD X-ray diffractomer using Cu Kα (1.54059 Å) radiation with the X-ray generator operating at 45 kV and 40 mA. TEM images were obtained on JEOL 2010 microscopes. The TEM sample was prepared by dropping a sample suspension in ethanol on a Cu grid coated with a carbon film. Selenium nanoparticles were prepared by the reduction of aqueous sodium seleno-sulphate solution with freshly prepared glucose solution. The particle size of identified Se-NPs were ranged from (50–60 nm) in diameter.
Experimental design
Thirty healthy male albino rats aged 21 days were divided into 6 groups (5 each). The rats of group (1) (negative control), were fed healthy feeds and water. While, in group (2) (positive control) rats were orally administered AFB1 at a dose of (50 µg/kg of b.w. /day) (Qian et al., 2013; Jean-Baptiste et al., 2017). The rats of group (3) were given Se-NPs orally at dose of (0.3 mg/ kg of body weight/day) (Partha et al., 2015). The animals of group (4) were orally administered AFB1 at a dose of (50 µg/kg of b.w. /day) + Se-NPs (0.3 mg/ kg of b.w. / day). Whereas, rats of group (5) were given Se-NPs orally at dose of (0.5 mg/ kg of b.w. /day). The rats of group (6) were given AFB1 at a dose of (50 µg/kg of b.w. /day) + Se-NPs orally (0.5 mg/ kg of b.w. /day). Each of the daily doses dissolved in olive oil and given orally to rats using a feeding stomach tube attached to a hypodermic syringe and the experimental study was extended for 3 weeks.
Samples collection
At the end of experiment blood was collected from all rats, left to clot and sera were separated for biochemical analysis. Then rats in all treated groups were sacrificed and liver tissues were collected and kept frozen for detection of AFB1 residues.
Serum biochemical studies
The measurements of liver enzyme’s activity were done as the methods of Reitman and Frankel (1957), serum urea according to Wybenga et al. (1971). TNF-α and IL-6 were determined using commercially available ELISA kits according to manufacturers’ instructions (R and D Systems, Minneapolis, Minnesota, USA). Total iron, total iron-binding capacity (TIBC), and utilized iron-binding capacity (UIBC) (Kunish and small, 1970), transferrin saturation % (Tietz, 1996). Hepcidin and erythropoietin (EPO) hormones were measured by rat ELISA Kit (Glory Science Co., Ltd, USA), Catalogue No: 30981 and 94783, respectively. While, the determination of protein and their patterns electrophoresis were monitored (Sonnen-Wirth and Jaret, 1980).
Detection of aflatoxin B1 residues in liver tissues according to the method recommended by AOAC (2000).
Statistical analysis
Results were expressed as mean ± SE. Data were analyzed by ANOVA followed by Duncan’s post hoc test for significant difference (p < 0.05). The statistical analysis was carried out using Statistical Package for Social Sciences Version 16 released in (SPSS, 2007).
Results and Discussion
In the present study, the obtained results in (Table 1) showed that the most predominant isolated mould from the examined animal feed. Herein, one hundred and twenty feed samples (yellow corn, wheat bran, hay, poultry feed, processed animal feed and silage, 20 of each) were subjected for mycological examination. Eighty-one percent (81%) of samples were contaminated with different species of fungi. Most of the examined wheat bran, processed animal feed and poultry feeds were contaminated with fungi (90%). Followed by samples of silage (80%), yellow corn (70%) and hay (65%) samples were contaminated with fungi. Whereas, A. flavus was recovered from poultry feed (55%), hay (50%), yellow corn (45%) and processed animal feed and wheat bran (40% in each) and from (20%) of silage samples. Similar findings to our results were detected by Hassan et al. (2017), who recovered A. flavus as the most common isolated mould from animal and poultry feeds. Also, Nooh et al. (2014), recovered Aspergillus spp. and Penicillium spp. from yellow corn and mould spores can disperse in the air with the wind.
Currently, of 50 tested A. flavus that recovered from feeds, 35 isolates were aflatoxigenic (70%) and produced aflatoxin B1 and B2 at average levels of (32.0±1.22:187.0± 3.71. ppb). The highest mean levels of aflatoxins were produced by A. flavus that recovered from poultry feed (187.0 ± 3.71ppb), processed animal feed (95.0± 2.41ppb), wheat bran (70 ± 4.50), yellow corn (55 ±1.50) and silage (37.0 ±0.00). While, the lowest level of AFs was detected in hay samples (32.0 ±1.22) (Table 2). Our findings came in accord with the results yielded by (Frisvad et al., 2006). While the use of contaminated feeds with fungi and aflatoxins in animal and poultry resulted in harmful significant adverse effects (Nayak and Sashidhar, 2010) and produced significant toxicity and have potential carcinogen (FAO/WHO, 2007). In our study, the aflatoxins residues were at the rates of (70%, 75%, 90%, 85%, 80%; 70%) in samples of (yellow corn, wheat bran, hay, poultry feed, processed animal feed; silage), respectively. While the mean levels of AFs were (0.10±0.032 ppm), (0.053±0.060 ppm), (0.16±0.010 ppm), (0.083±0.170 ppm), (0.117±0.020 ppm); (0.15±0.030 ppm) in the above samples, respectively (Table 3). All types of aflatoxins (B1, B2, G1, and G2) were detected in all positive feed samples and the detected levels were over the permissible limits (FDA, 2000) (20 ppb) and these feeds can be resulted in health hazard for human and animal. Hassan et al. (2008) reported that the mean levels of aflatoxins were (3.4 ± 0.1 ppm) in positive feed samples (30%). In developing countries, liver injury and cirrhosis are associated with aflatoxicosis (FDA, 2000) and prevent protein, DNA synthesis and causes cell apoptosis (Rotter et al., 1996).
In the present work, chemical synthesis and characterization of Se-NPs was undertaken. The particles were spherical and granular in shape and in crystalline form with 50-60 nm in size as observed by scanning electron micrograph (SEM) (Figure 1). Whereas, the UV-VIS absorption spectrum of Se-NPs was (200–550 nm.) and maximum absorption peak observed at wave length of 400 nm (Figure 2).
Similarly, (Atul et al., 2010) synthesized Se-NPs by chemical method and the particle size of prepared Se-NPs was (20-80 nm). Recently, Jay and Shafkat (2018) green biosynthesized of Se-NPs using natural plants and sodium selenite solution. It appears that nano-materials have the ability of drug delivery to target cells and have been used in the various biomedical applications in the modern world (Suri et al., 2007).
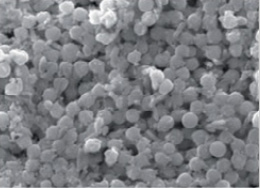
Figure 1: SEM analysis of the selenium nanoparticles showed the size and morphology (60 nm) at 9,500 magnifications.
Currently, the prepared Se-NPs were used ameliorating the induced hepatotoxicity by aflatoxicosis in male albino rats. After the end of experimental periods several biochemical parameters were monitored on all treated rats.
Concerning the effect of the AFB1 (Table 4), the present data revealed the significant increase in AST and ALT enzymes and decrease in urea level. These changes indicated the initial hepatocellular damage after AFB1 treatments (Choi et al., 2010) and the Kidney was one of the main targets of AFs and the way of its transformation (Li et al., 2018). Herein, the adverse effects of AFs on the hepatic and renal functions were also reported by Sun et al. (2015).
Currently, the tabulated results in (Table 5), showed an apparent decrease in levels of total protein, albumin, total alpha, total gamma and consequently total globulins as well as A/G ratio among rats exposed to aflatoxin B1. The binding of AFB1 with RNA and DNA of the cell resulting in confusion of transcription operation and prevention of their synthesis (Abu-Aita et al., 2008). Aflatoxins are an ,ß-unsaturated with electrophilic reactivity at the carbonyl carbon atom and could conceivably adduct amines, imidazole and sulfhydryl groups on proteins and enzyme via the Michael carbonyl condensation reaction can cause conformational changes that interfere with their function (Lee et al., 2010). The immune-toxic activity of aflatoxin resulted from degenerative alterations and cell death following necrosis and apoptosis (Al-Anati and Petzinger, 2006). Herein, the protein subfractions recorded a significant decrease this may ascribe to immunosuppressive effect of AFB1 (Table 5). These may be related to adverse effects of aflatoxin towered the hepatic cells and immune system (Yunus et al., 2011). The alteration in protein subfraction alpha-2, beta-2 and gamma-2 globulins may results of inflammatory tissue, increase in alpha-2 macroglobulin and beta-2 lipoprotein (Kaneko et al., (1997). Moreover, the hepatotoxicity induced by AFB1 was resulted in a marked elevation of TNF-α and IL-6 activities (Table 6). Similar findings were detected using a model of AFB1-induced liver damage (Aborehab and Waly, 2019). Also, AFB1 initiate production of reactive epoxide by cytochrome P450 enzyme in liver microsomal (Meissonnier et al., 2008). This causes oxidative stress, damages and apoptosis of liver cells and lipid peroxidation resulted in changes in cell permeability and the release of cytokines (Lawrence, 2009). Furthermore, AFB1 stimulated production of cytokines as interleukin-6 (IL-6), and tumour necrosis factor-α (TNF-α), mediate inflammation through interaction with the IL-6 receptor, and the TNF receptor (Hendrayani et al., 2016).
In our study, AFB1 resulted in decreased levels of serum iron, TIBC, UIBC and transferrin% compared with control (Table 7). While, the decrease in transferrin in rats fed AFB1 was attributed to a decrease in transferrin mRNA (Harris et al., 1998). The decrease in UIBC associated with AF-exposure, is probably resulted from the ability of AF- to induce decrease in the concentration of transferrin and β-globulin which responsible for iron binding capacity (Salem et al., 2007). Thus, AFB1 induced iron deficiency anemia which the common type of hypochromic-microcytic anemia. Similarly, the total iron concentrations were lowered in lambs fed AFB1 due to inflammation and protein metabolism (Edrington et al., 1994). While, Stoltzfus and Dreyfuss (1998) mentioned that the decrease in serum iron was due to the decrease in serum ferritin in animal fed AFB1.
Table 1: Prevalence of fungi in animal and poultry feeds.
Fungi | Yellow corn (20) | Wheat bran (20) | Hay (20) | Animal feeds (20) | Poultry feed (20) | Silage (20) | Total (120) | |||||||
% | No. | % | No. | % | No. | % | No. | % | No. | % | No. | % | No. | |
Total fungi | 70 | 14 | 90 | 18 | 65 | 13 | 90 | 18 | 90 | 18 | 80 | 16 | 81 | 97 |
Aspergillus flavus | 45 | 9 | 40 | 8 | 50 | 10 | 40 | 8 | 55 | 11 | 20 | 4 | 42 | 50 |
Aspergillus ochraceous | 15 | 3 | 0 | 0 | 0 | 0 | 10 | 2 | 15 | 3 | 10 | 2 | 8.3 | 10 |
Fusarium sp. |
5 | 1 | 0 | 0 | 5 | 1 | 10 | 2 | 15 | 3 | 10 | 2 | 7.5 | 9 |
Penicillium sp. |
10 | 2 | 0 | 0 | 25 | 5 | 25 | 5 | 10 | 2 | 15 | 3 | 14.1 | 17 |
Mucor sp. |
5 | 1 | 0 | 0 | 0 | 0 | 15 | 3 | 10 | 2 | 5 | 1 | 5.8 | 7 |
Candida albicans | 5 | 1 | 0 | 0 | 15 | 3 | 5 | 1 | 0 | 0 | 40 | 8 | 10.8 | 13 |
Rhodotorula sp. |
0 | 0 | 0 | 0 | 5 | 1 | 0 | 0 | 0 | 0 | 5 | 1 | 1.6 | 2 |
No.: Numbers of positive; %: Percent of positive / total.
Table 2: Aflatoxigenisity of isolated A.flavus from animal and poultry feeds samples.
Source of tested A. flavus |
Levels of produced aflatoxins (ppb) |
||||
Tested isolated A.flavus |
Mean levels ±SE | Types of AFs. | |||
% | No. of +ve | No. of tested | |||
Yellow corn | 77.7 | 7 | 9 | 55 ±1.50 |
AF B1, B2 |
Wheat bran | 62.5 | 5 | 8 | 70 ±4.50 |
AF B1, B2 |
Hay | 40 | 4 | 10 | 32 ± 1.22 |
AF B1 |
Animal feeds | 87.5 | 7 | 8 | 95 ± 2.41 |
AF B1, B2 |
Poultry feed | 100 | 11 | 11 | 187 ± 3.71 |
AF B1, B2 |
Silage | 25 | 1 | 4 | 37 ± 0.0 |
AF B1 |
Total | 70 | 35 | 50 | 32 ± 1.22: 187 ± 3.71 | - |
No.: Numbers; AFs: Aflatoxins.
Table 3: Prevalence of aflatoxins in animal and poultry feeds.
Types of feeds (20 of each) | % of +ve |
Levels of aflatoxins in samples (ppm) |
Types of AFs | ||
Mean ±SE | Min. | Max. | |||
Yellow corn | 70 | 0.10±0.032 | 0.032 | 0.12 |
B1,B2,G1, G2 |
Wheat bran | 75 | 0.053±0.60 | 0.024 | 0.16 |
B1,B2,G1, G2 |
Hay | 90 | 0.16±0.010 | 0.120 | 0.20 |
B1,B2,G1, G2 |
Poultry feeds | 85 | 0.083±0.170 | 0.160 | 0.35 |
B1,B2,G1, G2 |
Processed animal feeds | 80 | 0.117±0.020 | 0.100 | 0.22 |
B1,B2,G1, G2 |
Silage | 70 | 0.15±0.030 | 0.12 | 0.18 |
B1,B2,G1, G2 |
*The permissible limits of AF. Acc. FDA (2000) (20 ppb). Max.: Maximum; Min: Minimum; AFs: Aflatoxins.
Table 4: Serum levels of liver enzyme and urea (U/L) in the different experimental groups.
Parameters/ Enzymes |
G1: Control |
G2: AFB1 50 μg/kg b.w |
G3: Se-NPs 0.3 mg/kg b.w |
G4: 50 μgAFB1 + 0.3 mg Se-NPs/kg b.w |
G5: Se-NPs 0.5mg/ kg b.w |
G6: 50μgAF B1+ 0.5 mg Se-NPs /kg b.w |
ALT |
12.40±0.87d |
53.20±2.71a |
25.40±3.16c |
35.00± 4.43b |
14.60± 0.68d |
31.00± 2.61bc |
AST |
21.80±1.50c |
70.40±9.10a |
33.00±1.64bc |
37.60±2.84b |
24.20±1.50c |
30.00± 0.84bc |
Urea |
39.84±1.18ab |
34.15±1.24c |
43.63±1.34c |
37.49±1.98bc |
36.50± 0.90bc |
34.99±1.16c |
*Data are represented as mean value ±SE. n=5 male albino rats; *Values in the same raw with the different superscript letter are significantly different (P<0.05); * Selenium nanoparticles: Se-NPs, AFB1: Aflatoxin B1.
Table 5: Serum levels of patterns of protein electrophoresis fractions and subfractions (g/dl) in the different experimental groups.
Groups of treated animals / Parameters |
G1: Control |
G2: AF B1 50 μg/kg b.w |
G3: Se-NPs 0.3 mg/kg b.w |
G4: 50 μgAFB1 + 0.3 mg Se-NPs/kg b.w |
G5: Se-NPs 0.5mg/ kg b.w |
G6: 50μgAF B1+ 0.5 mg Se-NPs /kg b.w |
Total protein |
7.22±0.32a |
5.56±0.12c |
7.09±0.06a |
5.97±0.16bc |
6.88±0.08a |
6.27±0.22b |
Albumin |
2.50±0.06a |
2.04±0.04c |
2.42±0.05a |
2.20±0.04bc |
2.10±0.06cb |
2.23±0.07b |
Alpha1a |
0.45±0.03a |
0.34±0.02cb |
0.430±.01ab |
0.28±0.03c |
0.39±0.04ab |
0.42±0.05ab |
Alpha1b |
0.50±0.06a |
0.37±0.04a |
0.45±0.01a |
0.39±0.06a |
0.44±0.04a |
0.47±0.07a |
Alpha2 |
0.42±0.08a |
0.28±0.02b |
0.43±0.04a |
0.32±0.02b |
0.39±0.02ab |
0.42±0.03ab |
Total alpha |
1.38±0.15a |
0.990.06b |
1.31±0.04a |
0.990.07b |
1.22±0.06ab |
1.31±0.13a |
Beta1 |
0.56±0.09abc |
0.44±0.02c |
0.74±0.03a |
0.66±0.03ab |
0.70±0.02a |
0.72±0.08ab |
Beta2 |
0.76±0.02a |
0.58±0.02b |
0.62±0.01ab |
0.53±0.07abc |
0.64±0.04ab |
0.50±0.04bc |
T.beta |
1.32±0.08a |
1.01±0.03b |
1.37±0.04a |
1.19±0.10ab |
1.34±0.04a |
1.22±0.04a |
Gamma1 |
1.73±0.12b |
1.24±0.05c |
1.61±0.04b |
1.30±0.07c |
1.99±0.03a |
1.25±0.07c |
Gamma2 |
0.29±0.04b |
0.28±0.02b |
0.38±0.03a |
0.29 ±0.03b |
0.24±0.01b |
0.26±0.02b |
T.gamma |
2.02±0.13ab |
1.51±0.06c |
1.99±0.03b |
1.59 ±0.04c |
2.23±0.03c |
1.51±0.09c |
T.globulin |
4.72±0.26c |
3.52±0.14a |
4.67±0.02c |
3.77±0.15ba |
4.78±0.03a |
4.03±0.17b |
A/G ratio |
0.53±0.02ab |
0.58±0.03ab |
0.52±0.01b |
0.59±0.02a |
0.54±0.01ab |
0.55±0.02ab |
*Data are represented as mean value ±SE. n:3male albino rats; *Values in the same raw with the different superscript letter are significantly different (P<0.05).
Table 6: Levels of TNF-α and IL-6 (Pg/ml) in the different experimental groups.
Parameters |
G1: Control |
G2: AF B1 50 μg/kg b.w |
G3: Se-NPs 0.3 mg/kg b.w |
G4: 50 μgAF B1 + 0.3 mg Se-NPs/kg b.w |
G5: Se-NPs 0.5mg/ kg b.w |
G6: 50μgAF B1+ 0.5 mg Se-NPs /kg b.w |
TNF-α |
166.80±4.55c |
367.60±5.70a |
179.40±4.46c |
265.20±5.41b |
176.80±5.63c |
268.60±5.70b |
IL-6 |
31.00±0.71e |
46.07±0.59a |
36.20±0.37c |
34.80±0.37d |
34.40±0.40cd |
39.40±0.51b |
*Data are represented as mean value ±SE. n=5 male albino rats; *Values in the same raw with the different superscript letter are significantly different (P<0.05).
Table 7: Levels of serum iron profile (Iron, TIBC, UIBC (ug/dl) and Transferrin Saturation %) in the different experimental groups.
Parameters |
G1: Control |
G2: AF B1 50 μg/kg b.w |
G3: Se-NPs 0.3 mg/kg b.w |
G4: 50 μgAF B1+ 0.3 mg Se-NPs/kg b.w |
G5: Se-NPs 0.5mg/ kg b.w |
G6: 50μgAF B1+ 0.5 mg Se-NPs /kg b.w |
Iron |
114.90±1.08a |
80.47±0.67d |
115.40±1.12a |
94.06±0.44b |
117.09±1.20a |
89.02±0.65c |
TIBC |
175.60±0.93c |
150.20±0.80f |
184.20±0.37a |
167.40±0.68d |
180.80±0.73b |
162.60±0.75e |
UIBC |
175.60±0.93c |
69.73±0.97ab |
68.80±1.46b |
73.34±0.51a |
63.71±1.47c |
73.58±0.92a |
Transferrin saturation % |
65.45±0.90a |
53.58±0.49d |
62.65±0.72b |
56.19±0.20c |
64.77±0.74a |
54.75±0.44cd |
*Data are represented as mean value ±SE. n=5 male albino rats; *Values in the same raw with the different superscript letter are significantly different (P<0.05).
Table 8: Serum levels of Hepcidin and Erythropoietin (EPO) in the different experimental groups.
Parameter |
G1: Control |
G2: AF B1 50 μg/kg b.w |
G3: Se-NPs 0.3 mg/kg b.w |
G4: 50 μgAF B1 + 0.3 mg Se-NPs/kg b.w |
G5: Se-NPs 0.5mg/ kg b.w |
G6: 50μgAF B1+ 0.5 mg Se-NPs /kg b.w |
Hepcidin (µg/L) |
31.50±0.16c |
37.50±0.05a |
30.00±0.11d |
36.50±0.045b |
29.50±0.65d |
25.00±0.13e |
EPO (IU/L) |
4.15±0.18b |
3.70±0.12a |
6.00±0.07d |
4.00±0.16b |
6.25±0.26e |
5.70±0.18c |
*Data are represented as mean value ±SE. n=5 male albino rats; *Values in the same raw with the different superscript letter are significantly different (P<0.05).
Table 9: Levels of AFB1 residues in liver of rats in the different experimental groups (ppb).
Parameter |
G1: Control |
G2: AF B1 50 μg/kg b.w |
G3: Se-NPs 0.3 mg/kg b.w |
G4: 50 μgAF B1+ 0.3 mg Se-NPs/kg b.w |
G5: Se-NPs 0.5mg/ kg b.w |
G6: 50μgAF B1+ 0.5 mg Se-NPs /kg b.w |
AFB1 residues |
0.00 | 25.5±0.02 | 0.0±0.0 | 5.1 ±0.001 | 0.0±0.0 | 2.1±0.01 |
*The permissible limits of AFs. Acc. FDA (2000) (20 ppb).
In addition, there was a decrease in transferrin is a small protein and can be decreased with albumin in protein-losing disorders and low albumin and TIBC would be expected under these conditions (Williams and Enns, 1993a). The level of transferrin and transferrin receptor was monitored by Se-NPs and the total iron binding capacity (TIBC) was also ameliorated in treated groups (Murthy, 2013). Selenium nanoparticles have high bioavailability, low toxicity, high catalytic efficiency and a strong adsorbing ability (Rezvanfar et al., 2013). The supplementation of Se-NPs in foods caused antioxidant activity against the oxidative damages to liver cells due to chemical stress (Chen et al., 2013). The present results showed that, Se-NPs treatment along with AFB1 ameliorates AFB1-induced changes in protein contents in the serum of rats (Tables 4-7). This change due to the ability of Se-NPs to elevate protein and DNA synthesis (Cheng et al., 2003) and significantly decreased their damage in lymphocyte cells and also in bone marrow cells, which indicated the potential role of Nano-Se in protecting DNA damage. (Bhattacharjee, 2016). It plays an important role in the anemia of chronic inflammation through its relationship with the IL-6 that implicated the iron concentration (Roy and Andrews 2005). The Se-NPs have anti-inflammatory potential modulating pro-/anti-inflammation cytokine secretion profiles, and that the mechanism is partially due to inhibition of activations of NF-κB, JNK1/2 and p38 MAPKs (Wang et al., 2015). Se-NPs oral treatment increases the delayed hypersensitivity reaction and immune response (Yazdi et al., 2015).
Regarding, the tabulated results in (Table 8) showed that the treated animals with Se-NPs without administration of aflatoxin (G3 and G5) had a significant decrease in Hepcidin level in comparison with (G1). The comparison of our findings between (G4 and G6) with G2, the results showed a significant decrease in Hepcidin which is markedly obtained in (G6) aflatoxicated and treated with a high dose of Se-NPs. Indeed, involves a signal transducer and activator of transcription (STAT) site on the hepcidin promoter was evaluated by Verga-Falzacappa et al. (2007). The acute response to inflammations is regulated by the IL-6 in liver cells which caused activation of Janus kinases (Jak) that phosphorylate STAT3 in the nucleus and hence affected hepcidin gene expression (Wrighting and Andrews, 2006).
As a small peptide that does not seem to be connected to a large extent to the plasma proteins, hepcidin is eliminated from the plasma through the kidneys by ferroprotein-mediated endocytosis and proteolysis. Accordingly, concentrations of plasma levels of hepcidin may be increased in disease processes that reduce the hepcidin clearance from the kidneys (Zaritsky et al., 2009).
In this study, co-administration of nano-selenium to aflatoxin B1-treated rats was decreased hepcidin levels in G4 and G6 in comparison with G2. Nano-selenium suppresses the action of IL-6 through the down regulation of STAT3 activation (Verga-Falzacappa et al., 2007). Recently, Se-NPs supplementation caused elevation of antioxidant GPx activity and lower toxicity (Srivastava et al., 2014) and the induction of glutathione S-transferase and causes less oxidative stress (Gao et al., 2002). The serum EPO levels significantly decreased (P <0.5) in G2 in comparison with G1, G3 and G5. While, in G3 and G5 the level was significantly increased in comparing to G1. Moreover, the levels of erythropoietin in G4 and G6 were elevated than that of G2 with a highly significant increase in G6.
The significant decrease of erythropoietin level was observed in (G2) in comparison with G1, G3 and G5. Several studies showed that the erythroid colony formation in response to erythropoietin is impaired in the presence of pro-inflammatory cytokines (Kurzrock, 2001). This is related to the cytokines caused apoptosis induction, cell growth inhibition and dysfunction of erythropoietin receptor and iron metabolism damage (Morceau et al., 2009).
In another study, Macciò et al. (2005) found that pro-inflammatory cytokines decreased renal erythropoietin production and its activity in cells of other organs. Administration of nano-selenium to aflatoxin B1-treated rats (G4 and G6) showed increased EPO levels in comparison with G2. These findings may be due to the inhibitory effect of nano-selenium on proinflammatory cytokines which in turn improve the expression of EPO (Mohammed and Safwat, 2013). Herein, it is interesting to report here that all improvement in biochemical and hormonal parameters in treated aflatoxicated rats with Se-NPs were associated with successful degradation of aflatoxins residues in liver tissues. Herein, the current findings detected that the aflatoxin B1 residues in liver tissues decreased as the doses of Se-NPs increased (Table 9). Where, the aflatoxicated rats in G2 have detectable residues in liver tissues (25.5±0.02 ppb). While, the increase of Se-NPs dose to (0.5 mg/kg b.w.) in G6 resulted in more significant elimination of AFB1 from liver tissues (2.1 ±0.01 ppb) than that resulted from G4 which resulted in (5.1 ±0.001 ppb). Similarly, the supplementation of Se-NPs into foods and feeds have the ability to elevated the antioxidant activity of liver cells via improvement the immune status of the injured which increases the detoxification of the free radicals produced by aflatoxins (Chen et al., 2013).
CONCLUSION
Our foregoing fungal and mycotoxic pollutions of animal feeds results in significant losses in animal health and causes important burdens to the country’s economy regarding to meat, milk, wool and leather industries. The aflatoxicosis induces alterations in some biochemical, immunological and hormonal parameters related to anemia. As record in this direction, Se-Nps administration showed a significant protective effect against the adverse effect of aflatoxicosis in male albino rats. Hence, regular examination of animal feeds and their environment for fungi and mycotoxins contamination is a critical demand. Additional investigations are required to assure more safe doses of nanomaterials before field application in farm animals and poultry.
ACKNOWLEDGEMENT
The authors are gratefully acknowledged to Prof. Dr. Hazem Hassan Mahmoud, Laboratory of Elemental and Isotopic Analysis, Atomic Energy Authority, Egypt, for his kind assistance in the identification and characterization of the used selenium nanoparticles.
Author’s Contribution
Atef Hassan conceived and designed the experiments; Mogda K. Mansour, Rasha M. H. Sayed-Elahl, Hanan A. Tag El-Din, Mohamed E.A. Awad and Eman M. Younis did the methodology, All authors did manuscript preparation, analyzed the data and wrote this manuscript.Corresponding author: Rasha M. H. Sayed-Elahl. All authors read and approved the final manuscript.
Conflict of interest
The authors have declared no conflict of interest.
REFERENCES