Advances in Animal and Veterinary Sciences
Research Article
Detection of Gamma Interferon and Some Pro-Inflammatory Cytokines in SPF Chicks Experimentally Infected with Chicken Anemia Virus
Hanaa A. El-Samadony1, Hoda M. Mekky2*, Abeer S. Hafez3, Aalaa S.A. Saad1
1Poultry diseases department, Animal Health Research Institute, Dokki, Giza, Egypt; 2Poultry Diseases Department, Veterinary Research Division, National Research Centre, P.O. 12622, Giza, Egypt; 3Immunology Department, Animal Health Research Institute, Dokki, Giza, Egypt.
Abstract | Chicken infectious anemia is one of the most economic immunosuppressive problems facing commercial poultry sector worldwide. Present study demonstrated the viral load in body organs and viral specific antibody titres of the SPF chicks experimentally infected with chicken anemia virus (CAV) strain CAV/Kal.2 and its contact group in vivo. Also, followed the emergence of lysozyme activity and nitric oxide (NO) levels, pro-inflammatory {IL-1β, IL-6 and CXCLi2 (IL-8 like chemokine)}, type I IFN (IFN-α and IFN-β) and IFN-γ cytokines. The obtained data illustrated that CAV-specific antibody development started after 7 days post infection (dpi) and reached its maximum level at 21 dpi in infected group and contact group. The CAV genome was detected in tissues of the chicks of both infected and contact groups at 7 dpi and continued for 21 dpi with 7 days intervals between sampling. Lysozyme activity and NO levels were greatly impaired in CAV infected chicks. The relative mRNA expression levels of most examined cytokines in the infected group were increased on 14 dpi compared to 7 and 21 dpi. On the other hand, CXCLi2 was generally not altered by CAV infection. The contact group showed undetermined changes in all examined cytokines (IL-1β, IL-6, CXCLi2, IFN-α, IFN-β and IFN-γ). The obtained data revealed cytokine imbalances after infection with CAV as a result of hindrance of transcription of the most of the examined cytokines. As the immunosuppressive viruses of chickens may confuse with transcription for several cytokines (IL-1β, IL-6, IFN-α, IFN-β and IFN-γ), so we suggest using this confliction in order to evaluate the immune status of chickens.
Keywords | CAV, Pro-inflammatory cytokines, Infected SPF chicks, Lysozyme activity, Immunosuppressive viruses
Received | November 10, 2019; Accepted | February 26, 2020; Published | March 20, 2020
*Correspondence | Hoda M. Mekky, Poultry Diseases Department, Veterinary Research Division, National Research Centre, P.O. 12622, Giza, Egypt; Email: [email protected]
Citation | El-Samadony HA, Mekky HM, Hafez AS, Saad ASA (2020). Detection of gamma interferon and some pro-inflammatory cytokines in SPF chicks experimentally infected with chicken anemia virus. Adv. Anim. Vet. Sci. 8(4): 339-346.
DOI | http://dx.doi.org/10.17582/journal.aavs/2020/8.4.339.346
ISSN (Online) | 2307-8316; ISSN (Print) | 2309-3331
Copyright © 2020 El-Samadony et al. This is an open access article distributed under the Creative Commons Attribution License, which permits unrestricted use, distribution, and reproduction in any medium, provided the original work is properly cited.
INTRODUCTION
Chicken infectious anemia is an important worldwide disease in the poultry industry (Balamurugan and Kataria, 2006). Growth retardation, increased death rate, as well as vaccine failures and increased prevalence of secondary viral/ bacterial infections resulting from immunosuppression which are considered the main economic losses originated from the disease (Drén et al., 2000; Schat et al., 2011). The disease can be transmitted vertically and horizontally resulting in clinical and sub-clinical infections respectively (Yuasa and Yoshida, 1983).
All ages of both sexes of chickens can be infected by the virus (Schat and Van Santen, 2008). But, clinical signs may be manifested only from young chicks less than two weeks of age (Dhama et al., 2002; Schat, 2003). Characteristic clinical signs involve weakness, inappetance, unthriftiness, weight loss, paleness of comb, wattle, eyelids and legs (severe anemia) and sudden death (Kuscu and Gurel, 2008). Main lesions include changes in bone marrow color from red to pale to white and may possess a consistency of the fat (Todd and McNulty, 2007). All lymphoid organs involving thymus, bursa of Fabricius and spleen suffer from severe atrophy at the top stage of anemia. Also, the liver changes to pale than discolored and swollen. Areas of congestion and hemorrhages may appear in other visceral organs (Dhama et al., 2002).
The causative agent of the disease is chicken anemia virus (CAV) that related to family Circoviridae, genus Gyrovis (Schat, 2009). CAV is small about 25 nm in diameter, nonenveloped, icosahedral virus containing negative sense single-stranded circular DNA genome of 2.3 kb size (Yamaguchi et al., 2001).
The virus has acquired its importance as a result of owing unique characteristic features like highly contagious nature, stable and resistance nature, vertical transmission in addition to immunosuppressive potential (Bhatt et al., 2011). Viruses have progressed many subtle strategies for escaping immune supervision like interference with chicken IFN mRNA transcription (Giotis et al., 2015).
The virus targets the haematopoietic precursors in the bone marrow and thymic precursors in thymic cortex. It was reported that CAV infection particularly causes severe disorders in splenic T-lymphocyte functions causing weakened reactivity to phytohemagglutinin and concanavalina (non-specific mitogens) in addition to drop in interleukin production (Adair et al., 1991). In vivo or in vitro exposure to CAV has been associated with sever reduction in concentration and functions of macrophage (Cloud et al., 1992; McConnell et al., 1993a, 1993b).
Hosts typically respond to viral invasion by mounting complex, multifaceted, defensive immune responses that include substantial induction of type I IFNs (IFN-α and IFN-β), initial production of pro-inflammatory cytokines (IL-1β, IL-6 and CXCLi2 (IL-8 like chemokine), to drive Th1 responses with subsequent induction of the relevant Th1 cytokines, later production of anti-inflammatory cytokines to switch off the inflammatory responses after viral clearance to limit immunopathology and activation of apoptotic pathways. These are all accompanied by notable changes in gene expression. CAV is an immunosuppressive virus, and is thus thought to interfere in at least some of the host antiviral responses (Giotis et al., 2015).
This study demonstrated the viral load in body organs and viral specific antibody titres of the SPF chicks experimentally intramuscularly infected with CAV and its contact group. Also, followed the emergence of lysozyme activity and nitric oxide (NO) levels, pro-inflammatory (IL-1β, IL-6 and CXCLi2), type I IFN (IFN-α and IFN-β) and IFN-γ cytokines, of SPF chicks experimentally infected with CAV strain and its contact group.
MATERIALS AND METHODS
Virus strain
An Egyptian field isolate of CAV (CAV strain CAV/Kal.2; GenBank accession No. MH260568), kept in the Avian Virology unit, Poultry Diseases Department, Animal Health Research Institute, Dokki, Giza, was used for experimental infection purposes.
Experimental design
Seventy five specific pathogen free (SPF) chicks of one-day-old were divided into 3 equal groups (25 chicks/group) and directly treated as follow: Group 1, chicks were intramuscularly inoculated with 0.2 ml (log10 3.7 virus copies/ml) of CAV according to Hussein et al. (2001), Group 2, chicks were marked with leg bands and left as contact with group 1 at the same place and Group 3, chicks were kept as non-infected negative control. The experimental groups were housed separately in environmentally controlled isolation units and were given feed and water ad libitum till the end of experiment. At 7, 14 and 21 days post infection (dpi), 5 chicks/group were humanely euthanized for collection of blood and tissue samples. Blood were collected for serum separation to ELISA test, lysozyme activity and NO levels estimation. Liver, spleen, bone marrow and thyroid were collected for application of Quantitative real time polymerase chain reaction (qRT-PCR) to determine the viral copy number in the tissues of CAV-infected, contact and negative control chicks. Also, the mRNA expression levels of the cytokines viz., IL-1β, IL-6, CXCLi2, IFN-α, IFN-β and IFN-γ were evaluated in tissue samples.
Chicks in this study were handled in accordance with local laws and regulations by Ethical Committee for Medical Research at the National Research Centre, Egypt.
ELISA (Enzyme-Linked Immunosorbent Assay)
Serum samples were collected from chicks of negative control, virus-infected and its contact at 7, 14 and 21 dpi to monitor the development of CAV-specific antibodies using a commercially available CAV ELISA Kit (Biochek Lot No. BPT431).
Lysozyme activity
Lysozyme activity was determined as previously described (Schultz, 1987). Lysoplates were prepared by dissolving 1% agarose in 0.06 M PBS (pH 6.3) with 500 mg/L Micrococcus lysodeikticus. The agarose mixture was distributed into plates. 25 ul of serum samples and standard lysozyme were added to each well. After 18 h, the diameter of the cleared zones was measured and the lysozyme concentration was estimated from standard logarithmic curve.
Table 1: Primers used in the quantitative real time PCR assay for various cytokine genes and CAV
RNA target | primer sequence (5’-3’) |
28S | F: GGCGAAGCCAGAGGAAACT |
R: GACGACCGATTTGCACGTC | |
IFN-α |
F: GACAGCCAACGCCAAAGC |
R: GTCGCTGCTGTCCAAGCATT | |
IFN-β |
F: CCTCCAACACCTCTTCAACATG |
R: TGGCGTGCGGTCAAT | |
IFN-γ |
F: GTGAAGAAGGTGAAAGATATCATGGA |
R: GCTTTGCGCTGGATTCTCA | |
IL-1β |
F: GCTCTACATGTCGTGTGTGATGAG |
R: TGTCGATGTCCCGCATGA | |
IL-6 | F: GCTCGCCGGCTTCGA |
R: GGTAGGTCTGAAAGGCGAACAG | |
CXCLi2 | F: GCCCTCCTCCTGGTTTCAG |
R: TGGCACCGCAGCTCATT | |
CAV | CAV Q5:5'-GCCCCGGTACGTATAGTGTGAG-3' |
Cux-1* specific 5'-CCGTGAGAAAGATGACCCCTT-3' |
|
CAV probe 5' - (6FAM)-CTGCCGAACCCCCAATCTACTATGACTATCC-(TAMRA)-3' |
Nitric oxide (no) levels
100 µl serum samples were incubated at 25 ºC for 10 min with an equal volume of Griess reagent in flat bottom 96-well ELISA plates. The absorbance was measured at 550 nm using an ELISA plate reader and the NO concentration was calculated from a standard curve generated using NaNO2 (Rajaraman et al., 1998).
DNA/RNA tissue isolation
DNA/RNA Tissue isolation using PathoGene-spin™ DNA/RNA Extraction Kit for both viral loads in body organs and mRNA expression levels of the cytokines.
The collected organs have been homogenized in saline containing 2000 iu/ml Penicillin and 200 mcg/ml Streptomycin. These organs were ground making homogenized (20% W/V) then centrifuged at 3000 rpm for 15 minutes. After centrifugation the clear supernatant fluid was kept at 20°C (Mc Nulthy, 1998). Tissue suspensions supernatants were treated with the PathoGene-spin™ DNA/RNA Extraction Kit following the manufacturer’s instructions (iNtRON Biotechnology, Seongnam, Korea). After measuring DNA concentrations using the NanoDrop ND-1000 (NanoDrop, Wilmington, DE) the samples were stored at -70°C.
Complementary-DNA
The RNA extract was mixed with a master mixture of reverse transcriptase RT buffer and incubated at 42°C for 15 min, followed by further incubation at 85 °C for 5 sec to inactivate the reverse transcriptase. The synthesized oligonucleotide primers (metabion international AG) used in this study were illustrated in Table 1.
Quantitative real time pcr for virus load and cytokine mRNA expression level
The pool from collected organs (liver, spleen, thymus and bone marrow) from all groups of experiment at 7, 14 and 21-dpi were submitted to the quantitative real-time PCR examination to calculate the number of copies of each CAV strain after infection in SPF chicks.
Quantification of the viral DNA in the various tissues: Oligonucleotide primer sequences were used for the detection of chicken anemia virus DNA using real-time Taqman assays (Table 1). Viral load was determined using previous formed standard curve (El-samadony et al., 2019).
The reactions were carried out in an AB Applied Biosystems. The conditions consisted of 95 °C for 10 min then 40 cycles consisting of 95 °C for 15 sec and 60 °C for 1 min. The real-time PCR data acquisition and analysis were performed using Analysis computer system, V 2.2.2 software (AB Applied Biosystems) (Markowski-Grimsrud et al., 2002).
mRNA expression levels of IL-1β, IL-6, CXCLi2, IFN-α, IFN-β and IFN-γ: The cDNA samples of the tissue were subjected to real time PCR analysis (primers in Table 1) using aV2.2.2 software (AB Applied Biosystems). Duplicate sets of each reaction sample were with the following cycle profile: one cycle of 48°C for 30 min and 95°C for 20 sec, followed by 40 cycles of 95°C for 3 sec and 60°C for 30 sec.
Statistical analysis
All the values obtained in the study were represented as mean±SD. Viral copy numbers for tissue sample were interpolated from the generated standard curve. For cytokine expression levels, values were normalized to the endogenous control (28S) and the fold changes in the target genes were determined using the 2-ΔΔ Ctmethod of Livak and Schmittgen (2001). The results of lysozyme and nitric oxide assays were statistically analyzed using one-way ANOVA test on a computer program SPSS-14 according to Norusis (2006).
RESULTS
Anti-cav antibody levels in serum and viral load in body organs
The results of ELISA examined serum which collected from the infected and its contact SPF-chicks for CAV strains CAV/Kal.2 were summarized the GMT (Geometric Mean Titer) in Figure 1. CAV-specific antibody development started after 7 dpi in the chicks of the virus-infected group and its contact, reached its maximum level at 21 dpi in groups (infected and its contact). The results of ELISA examined serum of negative control group were negative during all test period.

Figure 1: Comparison between the CAV-specific antibody titre in the serum and the virus load in tissue. The virus-specific antibodies were detected using ELISA.
The CAV genome was detected in all chicks of infected group and its contact at 7 dpi with seven-day intervals up to 21 dpi (Figure 1). Highest level of CAV genome was observed at 7 dpi (log10 5.36±0.54) in tissues of infected group, but highest level of CAV genome in tissues of contact group was at 14 dpi (log10 3.7±0.66). While lower level of CAV genome in tissues were log10 4±46 in infected group at 14 dpi and the viral genome wasn’t detected at 7 dpi in tissues of contact group.
Lysozyme activity and nitric oxide (no) levels
The obtained results of lysozyme level (Figure 2) revealed significant and very prominent decrease in lysozyme concentration in infected group (group 1) with mean value (4±0.9) compared to control group (group 3) with mean value (12.2±2) at 14 dpi.
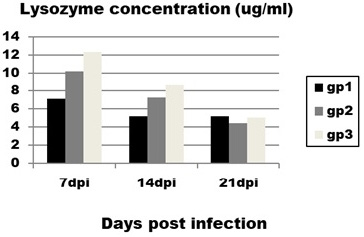
Figure 2: Effect of CAV infection on lysozyme concentration. Results expressed as mean±standard error.
Regarding to NO concentration (Figure 3) it is obvious that infected birds (group 1) showed significant decrease in concentration of NO at 7 and 14 dpi with mean value (7.1±0.7 and5.2±0.19 ) compared to control group (group 3) with mean value (12.3±0.6 and 8.6±1.4) at the same intervals.
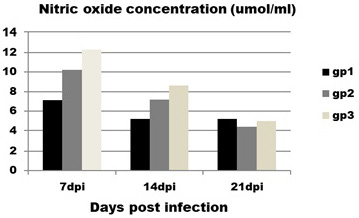
Figure 3: Effect of CAV infection on nitric oxide (NO) concentration. Results expressed as mean±standard error.
Contact group (group 2) does not show any significant differences with infected and control groups at all intervals.
Cytokine mRNA expression levels
Expression levels of transcripts for the signature pro-inflammatory (IL-1β, IL-6 and CXCLi2), type I IFN (IFN-α and IFN-β) and IFN-γ cytokines were measured by qRT-PCR (Figures 4 and 5). In the infected group the relative mRNA expression levels of examined cytokines were increased on 14 dpi compared to 7 and 21 dpi intervals, except CXCLi2 which was generally not altered by CAV infection in the infected group. However undetermined changes in contact group in examined cytokines were found.

Figure 4: Effect of CAV infection on cytokine expression in the examined tissues of sacrificed birds on various post-infection days in the infected group.

Figure 5: Effect of CAV infection on cytokine expression in the examined tissues of sacrificed birds on various post-infection days in the contact group.
DISCUSSION
Clinical disease is generally observed in young chicks (one to three weeks) which are infected either vertically or horizontally (Goryo et al., 1989a, 1989b; Smyth et al., 1993; Adair, 2000).
It was found CAV genome in examined tissue (pool of thymus, bone marrow, spleen, bursa and liver) at 7 dpi, this result is consistent with a study by Giotis et al. (2015) who reported that CAV antigen is first detected in thymus and bone marrow at 4–7 days post inoculation in 1-day old infected chicks. This is attributed to that CAV, like many other viruses obstructs/evades host cellular antiviral processes and exploits the host cellular resources to produce viral gene products. Hoop and Reece (1991) and Smyth et al. (2006) mentioned that the virus spreads by viraemia, CAV genome can be detected in cells of the bone marrow, thymus and spleen at three to four dpi. As observed high viral copies at 7-days in infected group and there was also gradual increase in detectable ELISA antibody titer at the same age, this was as a result of development of virus-neutralizing antibodies which normally developed within 1 to 2 weeks (Snoeck et al., 2012; Van Santen et al., 2004). However, comparing the levels of antibody detected by ELISA at 21 dpi among individual chickens in infected group and contact group these findings matched with result found by Tongkamsai et al. (2019) who detected the anti CAV antibody titre at 7dpi and the gradual increasing in antibody titre until 21dpi. Joiner et al. (2005) found that higher virus levels corresponded to higher antibody levels, suggesting that higher antibody levels were a result of greater stimulation by virus. Adair (2000) also approved that B-cells appear unaffected by the CAV, although the significantly higher anti-CAV antibody titres observed on 21 dpi, still higher viral concentrations in the tissue were found. Such observations indicate other immune components are also responsible for complete recovery (Wani et al., 2014).
Lysozymes perform many roles, including in host defense and innate immunity pathways. Lysozyme not only has antibacterial activity but also antiviral, anti-inflammatory, anticancer and immunemodulatory activities (Sava, 1996). Lysozyme is highly distributed in the organisms, being lysosomes of monocytes, macrophages and granulocytes (Gill, 1995). On the other hand, NO has been proposed as a host anti-microbial system (Akaiker and Maeda, 2000). Production of Nitric oxide is initiated in by inducible nitric oxide synthases (iNOS) in activated macrophages and neutrophils during defense and immunological reactions (Salleh et al., 2015).
The decreased level of lysozyme and NO concentration in CAV infected chicks may be attributed to the evidence that CAV infection has effect on immunological profile of the young chicks and reports the down regulatory changes in granulocyte macrophage-colony stimulating factor (GM-CSF) in chicks with younger ages of up to 2 weeks (Basaraddi et al., 2013).The results also agree with Wani et al. (2016) where they found that CAV infection significantly decrease all haematological parameters at different post infection include packed cell volume (PCV), total leukocyte count (TLC), peripheral lymphocyte count (PLC) and peripheral heterophil count (PHC).
In our study, the immune-related analysis of the transcriptional response pro-inflammatory (IL-1β, IL-6 and CXCLi2), type I IFN (IFN-α and IFN-β) and IFN-γ, of host cells to CAV in vivo was estimated by qRT-PCR. In infected group, at 7 dpi in examined tissue, there was proof of stimulation of an innate immune response, as mRNA expression levels of the pro-inflammatory cytokines (IL-1β and IL-6) and the type I IFNs (IFN-α and IFN-β), were up-regulated (between 1- and 2-fold). These results were compatible to results reported by Wani et al. (2014) who found that IL-1β and IFN-γ were increased (between 3- and 5-fold). Although, these induction levels were lower than those noticed for other immunosuppressive viral infections, such as infectious bursal disease virus (IBDV) (Eldaghayes et al., 2006) and Marek’s disease virus (MDV) (Kaiser et al., 2003). As after IBDV infection, mRNA expression levels of IL-1β and IL-6 were increased 10-fold and 25-fold, respectively. Induced innate responses after viral infection in the chicken usually comprise also up-regulation of the IL-8-like chemokine, CXCLi2 (Eldaghayes et al., 2006; Kaiser et al., 2003). Our results revealed slight down-regulation for mRNA expression of CXCLi2 at 14 dpi. Usually, induced anti-viral innate response would cause the production of IFN-γ cytokine, starting from about 5 dpi and peaking up to 14 dpi. In this study, following CAV infection, IFN-γ expression was up-regulated 2-fold at most. Similar results obtained by Wani et al. (2014) and Giotis et al. (2015). Although, IFN-γ was increased by about 250-fold and 25-fold following IBDV infection (Eldaghayes et al., 2006) and MDV infection (Kaiser et al., 2003), respectively. At 21 dpi, all cytokines were showed dramatically down regulating in the infected group, this occurred as a result of decreased IFN production by mitogen-stimulated splenic lymphocytes in vitro between 21- and 29- dpi (Bassami et al., 1998; Biagini, 2011; McConnell et al., 1993b). Normally, for a viral infection in the chicken, such an induced innate role (prolonged for up to two weeks post infection) in response against virus decrease after induction of a Th1-dominated adaptive immune response.
In contact group, immune-related analysis of the transcriptional response (pro-inflammatory (IL-1β, IL-6 and CXCLi2), type I IFN (IFN-α and IFN-β) and IFN-γ), of host cells to CAV in vivo was very low or neglected this may be due to low amount of virus through infection and viral load in tissues. Also, lysozyme and nitric oxide were not affected. Such results are agreed with Wani et al. (2016) where he established that, vertical transmission of CAV in field are associated to cause a more severe disease than chicks infected experimentally at day old age as there are more chances of complications by co-infections.
The present data indicate an immunosuppression of chicks during infection. Impairment of the immune response by CAV infection may result directly from damage to hematopoietic and lymphopoietic tissues and subsequent generalized lymphoid depletion and perhaps also from cytokine imbalances.
CONCLUSION
The obtained data revealed cytokine imbalances after infection with CAV as a result of hindrance of transcription of the most of the examined cytokines. As the immunosuppressive viruses of chickens may confuse with transcription for several cytokines (IL-1β, IL-6, IFN-α, IFN-β and IFN-γ), so we suggest using this confliction in order to estimate the immune status of chickens.
AUTHORS CONTRIBUTION
All authors contributed equally.
Conflict of interest
Authors declare that there is no conflict of interest.
REFERENCES