Advances in Animal and Veterinary Sciences
Research Article
Evaluation of the Modulatory Effects of Copper Salts on the Process of Angiogenesis (Neovascularization) with Therapeutic Perspectives
Rekha Khandia1, Pratibha Vishwakarma1, Abhinav Dwivedi1, Reena Mehra1, Anshumala Kujur1, Kuldeep Dhama2, Ashok Munjal1*
1Department of Genetics, Barkatullah University, Bhopal-462 026 (M.P.), India; 2Division of Pathology, Indian Veterinary Research Institute, Izatnagar, Bareilly-243 122 (Uttar Pradesh), India.
Abstract | Angiogenesis is a vital physiological process of formation and development of new blood vessels (neovascularization) from pre-existing ones, essential for embryonic development and growth especially in the growing stages. Copper (Cu) is known to promote angiogenic factors like VEGF (Vascular Endothelial Growth Factor), FGF (Fibroblast growth factor), angiopoitin and others, and as per previous reports, depending upon the material, Cu sometimes inhibit angiogenesis. We investigated the effects of different Cu salts (Copper chloride, copper acetate, alkaline copper tartrate and copper carbonate) on the process of angiogenesis among chicken chorioallantoic membrane (CAM) model by assessing histopathological alterations. Out of the four Cu salts studied, copper carbonate was found to be pro-angiogenic and did not reveal any detrimental effect, and therefore can have potential to be explored as a therapeutic material under health conditions requiring neovascularization. Rest other Cu salts (Copper chloride, copper acetate and alkaline copper tartrate) investigated were anti-angiogenic and induced inflammation in experimental material. Observing the importance of Cu in neovascularization, its salts can be used in therapeutic strategy either for treating disorders caused by excess angiogenesis or reduced angiogenesis.
Keywords | Angiogenesis, Neovascularization, Endothelial cells, Chorioallantoic membrane, Copper salts, Therapy
Editor | Yashpal S. Malik, Indian Veterinary Research Institute, Uttar Pradesh, India.
Received | June 30, 2016; Accepted | July 12, 2016; Published | July 22, 2016
*Correspondence | Ashok Munjal, Department of Genetics, Barkatullah University, Bhopal-462 026 (M.P.), India; Email: [email protected]
Citation | Khandia R, Vishwakarma P, Dwivedi A, Mehra R, Kujur A, Dhama K, Munjal A (2016). Evaluation of the modulatory effects of copper salts on the process of Angiogenesis (Neovascularization) with therapeutic perspectives. Adv. Anim. Vet. Sci. 4(8): 405-410.
DOI | Http://dx.doi.org/10.14737/journal.aavs/2016/4.8.405.410
ISSN (Online) | 2307-8316; ISSN (Print) | 2309-333
Copyright © 2016 Khandia et al. This is an open access article distributed under the Creative Commons Attribution License, which permits unrestricted use, distribution, and reproduction in any medium, provided the original work is properly cited.
INTRODUCTION
The term ‘angiogenesis’ refers to formation and development of new blood vessels (neovascularization) from the pre-existing ones (Birbrair et al., 2014; 2015). It is a multi-step and complex physiological process, essential for development (Yoo and Kwon, 2013), growth (Van Lessen et al., 2015) and wound healing (Johnson and Traci, 2014). Both excessive and insufficient angiogenesis conditions are responsible for pathological interventions. Excessive angiogenesis is associated with disorders like diabetic retinopathy (Praidou et al., 2010), cancer (Dudek et al., 2012), arthritis (Elshabrawy et al., 2015), age related macular degeneration (Ng and Adamis, 2005) etc. Insufficient blood supply in case of reduced angiogenesis imposes the risk of tissue death in several diseases such as ischemic chronic wound (Frykberg and Banks, 2015) and coronary artery diseases (Kastrup, 2010). It is also required for bone integration and survival in fracture repair (Saran et al., 2014).
Copper (Cu) is supposed to have pro-angiogenic activity owing to activation of pro-angiogenic growth factors and promoting endothelial cell migration, growth, and tube formation (Saghiri et al., 2015). It stimulates various factors viz. vascular endothelial growth factor (VEGF), fibroblast growth factor-2 (FGF2), tumor necrosis factor (TNF)-α and platelet-derived endothelial cell growth factor (PD-ECGF), which are responsible for pro-angiogenic effects (Gupte and Mumper, 2009). It is also required for the activation and expression of hypoxia-inducible factor-1 (HIF-1), a major transcription factor, responsible for regulating the expression of VEGF (Xie and Kang, 2009). Cu is also associated with cell migration, invasion, proliferation and the formation of tubular structures (Gao and Xu, 2008).
Copper or copper salts have been reported to increase angiogenesis among several model systems viz. bovine aorta endothelial cell system (McAuslan et al., 1983), rabbit corneal system (Raju et al., 1982), in vivo (Alessandri et al., 1984; Sanaat et al., 2011; Mroczek-Sosnowska et al., 2015) etc. Cu in the form of salt (CuSO4) or nanoparticles, when introduced into chicken embryos did not interfere with embryonic growth or development, but has been shown to increase angiogenesis via increasing the mRNA of activators like VEGF and FGF (Mroczek-Sosnowska et al., 2015). Recently, in few reports, contradictory to previous findings, Cu has been found to be anti-angiogenic too (Chang et al., 2015; Gandin et al., 2015). Hence, it necessitates evaluating different Cu salts for defining their pro-angiogenic or anti-angiogenic effects. The present study aimed to elucidate the angiogenic modulating effects of four Cu salts [Copper chloride, CuCl2; copper acetate, Cu (CH3COO)2; alkaline copper tartrate, C4H4CuO6; and copper carbonate, CuCO3] on chorioallantoic membrane (CAM) model of chicken.
MATERIALS AND METHODS
The four different Cu salts [CuCl2, Cu(CH3COO)2, C4H4CuO6 and CuCO3] were used in the present study. Their effects on the process of angiogenesis were evaluated using the chicken chorioallantoic membrane (CAM) model (Ribatti et al., 1997; Wierzbicki et al., 2013). The embryonated chicken eggs (n = 50) of 9 to 11 days of age, weighing around 61±3 g were procured from State poultry farm, Raisen Road, Bhopal (MP). The eggs were divided into five experiments groups (10 eggs in each), comprising of one control group (Group I) and four treatment groups (Group II- treated with copper chloride, Group III- treated with copper acetate, Group IV- treated with alkaline copper tartrate and Group V- treated with copper carbonate).
The egg surfaces were cleaned and wiped with 70% ethanol. A total of 200µl of 0.5M of each Cu salts [CuCl2, Cu(CH3COO)2, C4H4CuO6 and CuCO3] were directly introduced onto the CAM of respective group of embryonated eggs using 26 gauge hypodermic syringe along with antibiotic (Ampicillin 50 µg/ml, Streptomycin 10 µg/ml) and antimycotic solution (Amphotericin 10 µg/ml). Control group received phosphate buffered saline (PBS) with antibiotic and antimycotic solution. The eggs were resealed with cellophane tape and incubated for 72 hrs in a humid incubator chamber at 37°C. The eggs were then opened to observe the effects of different copper salts for angiogenesis modulation. The CAM was observed and further it was harvested and subjected to histopathological analysis using Hematoxylin and Eosin stain.
RESULTS
The embryonated chicken eggs of all the five experimental groups were examined/ opened after 72 hrs of incubation after treatment with different copper salts. Modulatory effects of different Cu salts on the process of angiogenesis by visualizing the gross and histopathological alterations in chorioallantoic membranes (CAMs) were observed.
The CAM of control group (Group I) revealed well arbourized vascular system, with several major and minor blood vessels (Figure 1A). In the case of copper chloride (Group II) and copper carbonate (Group V) treated groups, the blood vessels were observed as thin with less number of branch points (Figure 1B). In copper acetate treated group, CAM had more number of branch points with well noticed arbourization. Among alkaline copper tartrate treated group, CAM was firmly sticked with chalaza having less number of branch points and blood vessels.

Figure 1: (A) CAM of control eggs (Group I) with well arbourized vascular system; (B) CAM of copper chloride treated eggs (Group II) showing thin blood vessels with less number of branch points
Histopathology of the control (Group I) revealed normal thickness of CAM, with concordant dispersed mesodermal blood vessels (BV) and vein (V) with loose connective tissue (Figure 2A). Whereas, copper chloride treated CAM (Group II) exhibited reduced size and number of major blood vessels, with dense fibroblast (FB) cells (Figure 2B). Copper acetate treated CAM (Group III) revealed uneven thickness, reduced thickness of allantoic epithelial membrane (AE), less number of major and minor blood vessels with densely accumulated fibroblast (FB) cells, indicative of inflammation in the tissue (Figure 2C). Among alkaline copper tartrate treated group (Group IV), irregular thickness of CAM, with reduced thickness of chorionic epithelium (CE) and dense fibroblast accumulation was observed (Figure 2D).
It has been observed that groups treated with copper acetate (Group III) and copper tartrate (Group IV) demonstrated the inverse patterns in reduction in thickening of AE and CE. However, copper carbonate treated (Group V)
(A) Control CAM showing mesodermal LBV with SC and AE and CE of equal thickness (B) Copper chloride treated CAM showing reduced thickness in CE (C) Copper acetate treated CAM showing hypodermic LBV and FB cells (D) Copper tartrate treated CAM showed thin AE, but no blood vessel and densely accumulated FB cells (E) Copper carbonate treated CAM showed numerous BV with SEC, indicative of pro-angiogenic activity [LBV (Large Blood Vessels); BV (Blood Vessels); V (Vein); AE (Allantoic Epithelium); CE (Chorionic Epithelium); FB (Fibroblast); SEC (Sub Epidermal Capillary plexus); SC (Small Capillary)]
CAM was different from the other four groups, exhibiting large number of blood vessels (BV) of mesodermal origin (Figure 2E), which further converge to epidermis and formed sub-epidermal capillary (SEC) plexus, indicative of pro-angiogenic activity. It also did not alter normal morphology of CAM and unlike other treatment groups, did not caused tissue inflammation.
DISCUSSION
Angiogenesis is a vital process of growth and development and it is a delicate balance between pro-angiogenic and anti-angiogenic substances. Tissues get proper nourishment through the network of blood vessels, both excessive and deprived angiogenesis leads to pathological conditions. Gold (Arvizo et al., 2011) and silver (Khandia et al., 2015) nanoparticles are known to inhibit angiogenesis, whereas copper nanoparticles have been shown to have pro-angiogenic effect (Mroczek-Sosnowska et al., 2015). Cu acts as a stimulator of angiogenesis, vasculogenesis and endothelial cell migration by expressing angiogenic growth factors like VEGF, FGF and TNF (Finney et al., 2009). Angiogenin, a strong blood vessel formation inducer, binds to endothelial cell receptors to form new blood vessels, with 4.8 folds greater affinity in presence of Cu2+ (Soncin et al., 1997). Both, in form of Cu salt (CuSO4) and metallic Cu (CuO), it exhibited pro-angiogenic activity (Mroczek-Sosnowska et al., 2015). After applying on HUVECs (Human Umbilical Vein Endothelial Cells), the alloy of Cu with Mg revealed more number of branch points and a more complex morphology of angiogenesis (Liu et al., 2016).
On one side, Cu is increasing angiogenesis, whereas on the other side, some Cu compounds are known to have anti-angiogenic activity. Chang et al. (2015) revealed that CuO nanoparticles down-regulated the expression of vascular endothelial growth factor (VEGF) and VEGF receptors in endothelial cells. Homoleptic phosphino copper (I) complexes also repressed angiogenesis by inhibiting the fundamental steps of blood vessel formation like endothelial cell adhesion, migration, alignment, protease secretion and tubule formation (Chang et al., 2015; Gandin et al., 2015). The reason behind the contradictory behaviour can be explained on the basis of nature of material used for study, like solubility, ionic strength, dissociation constant and pH change in microenvironment surrounding the affected area.
Therefore, it became important to evaluate several Cu compounds for their pro- or anti-angiogenic activity. Locally available Cu levels are found to regulate the growth or regression of new blood vessels (Mroczek-Sosnowska et al., 2015). The extracellular Cu may directly bind to endothelial cell receptors and in turn it can stimulate a number of intracellular signaling pathways including PI3K (phosphoinositide 3-kinase)/ Akt, which is associated with up-regulation of survivin (apoptosis inhibitor) in endothelial cells and protection of endothelium from death-inducing stimuli (Papapetropoulos et al., 2000). Cu transporter proteins i.e. copper-transporting ATP7A and 7B (ATPase α-polypeptide and β-polypeptide) and high affinity copper uptake protein (Copper transporter 1, CTR1), help in maintaining homeostasis of copper (Singleton et al., 2010).
The uptake of Cu by CTR1 is temperature and pH dependent (Lee et al., 2002). However, the exact mechanism of transport is unknown. Here in the present study, we investigated four Cu salts (the pH order was CuCl2 < Cu(CH3COO)2< CuCO3 < C4H4CuO6); and used alkaline copper tartrate as very basic (pH 10.0), and copper chloride as acidic (pH 4.0). Therefore, it can be assumed that these solutions must have created a microenvironment, which was not suitable for proper functioning of CTR1 and also the pH adversely affected the CAM cells and hence inflammation occurred. The same also resulted in the induction of dense fibroblast accumulation with reduced vascularization in copper tartrate and copper chloride treated CAM. Our results are in agreement with the earlier studies of Badarau and Dennison (2011); demonstrated that ATP7A transporters are dependent upon pH and the transporter affinity is decreased approximately 5-fold, when the pH was lowered from 7-8 to 6.1. The acidic pH alters the status of charge onto the amino acids present in transporter proteins. Acidic pH could alter the charge status of the amino acids, for example, histidine residue of ATP7A activated tyrosinase in the active site, where charge alteration might modify the incorporation of the metal ion into active site and inappropriate incorporation could be assumed (Bin et al., 2015).
In a zebra fish model, after exposure, the accumulation of Cu nanoparticles (CUO) and CuCl2 in zebra fish larvae and ZFL (zebrafish cell line) was compared, despite using high nanoparticle concentration (5:1 in in vitro and 3:1 in vivo, respectively) the degree of accumulation of Cu was not much different (Chang et al., 2015). It indicated that accumulation is not dependent on extracellular Cu concentration. Therefore the deciding factor remains the affinity of Cu with ion transporter proteins, which in turn is dependent on dissociation of Cu compounds to release Cu ions and surrounding pH. Copper carbonate (pH 6) and copper acetate (pH 6.5), should efficiently bind to the transporter, but copper acetate (pH 6.5) also revealed inflammation and angiogenesis inhibition due to unexplained reasons. Copper carbonate (pH 6) showed pro-angiogenic activity, as it did not affected ATP7A transporter activity and Copper accumulated in cytosol might have helped in expressing pro-angiogenic factors like VEGF, FGF and angiopoitins, resulted in increased angiogenesis (Figure 3). Hence, copper carbonate can be used as pro-angiogenic material.
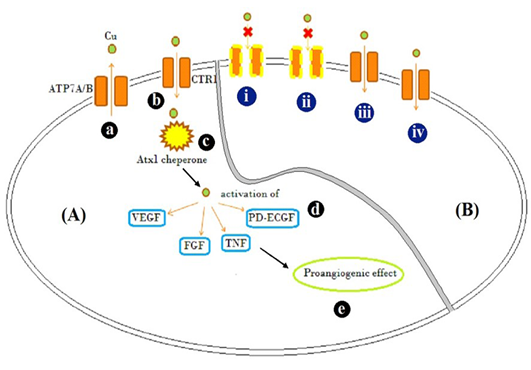
Figure 3: Schematic representation
Schematic representation of [A] Mode of action of Cu traffic; (a) ATP7A/ B are Cu transporter proteins regulating intracellular Cu concentration (b) CTR1 is Cu uptake protein (c) Atx1, a Cu uptake protein which is responsible for intracellular Cu binding and trafficking and release of Cu activates (d) VEGF, FGF, TNF and PD-ECGF growth factors (e) resulting in pro-angiogenic effect. [B] Illustration of possible mechanism by which different Cu salts altered angiogenesis and tissue patho-physiology (i) CuCl2, (acidic) and (ii) C4H4CuO6 (basic), possibly inactivated copper transporters by altering the net charge on them and showed inflammatory response evident by accumulation of fibroblast cells (Figure 2B and D) (iii) Cu(CH3COO)2 resulted in Cu transport mediated anti-angiogenesis, but caused inflammation too (Figure 2C) (iv) CuCO3 allowed entry of Cu and acted as pro-angiogenic material
Currently, many pathological conditions like rheumatoid arthritis, chronic wound and coronary artery diseases etc., which arose from the reduced angiogenic condition, are treated with pro-angiogenic therapies. Copper carbonate could be a promising pro-angiogenic material and need to be evaluated further to be used as therapeutics.
ConclusIONs
Cu in form of salt (CuSO4), metallic Cu (CuO), Cu alloy (Cu-Mg) and Cu nanoparticle, has shown to modulate angiogenic activity. It has shown both the sides of the coin, pro-angiogenic as well as anti-angiogenic activities; hence it is essential to evaluate different Cu compounds to observe which compound reveal what kind of activity. Here in the present study, copper acetate, copper chloride, and alkaline copper tartrate behaved as anti-angiogenic compounds, but caused inflammation in the CAM tissue, hence are not good for anti-angiogenic treatment. Copper carbonate, exhibited pro-angiogenic effect, with no detrimental effect on tissue and therefore could be a potential candidate for therapies requiring increased angiogenesis. Further the pro-angiogenic activity of copper carbonate may be tested in complex model systems (viz. rat corneal assay) or experimentally induced chronic wound or rheumatoid arthritis among animals.
ACKNOWLEDGMENTS
Financial assistance from DBT-IPLS is gratefully acknowledged. Authors of the manuscript thank and acknowledge their respective Universities/ Institutes.
CONFLICTS OF INTEREST
The authors declare that no conflict of interest.
AUTHOR’S CONTRIBUTION
Pratibha Vishwakarna and Anshumala Kujur executed the experimental work, Abhinav Dwivedi and Reena Mehra assisted in histology and collection of literature, Rekha Khandia and Ashok Munjal designed the study, interpreted the results and written the manuscript, Kuldeep Dhama reviewed the manuscript.
REFERENCES