Advances in Animal and Veterinary Sciences
Research Article
Pharmacokinetic Interactions between Intravenous Ciprofloxacin and Oral Ferrous Sulfate
Orooba Mohammed Saeed Ibrahim*, Ali Farid Shakir
Department of Physiology and Pharmacology, College of Veterinary Medicine, Baghdad University, Iraq.
Abstract | The changes in the bioavailability of oral antimicrobial activity in in vitro focus of many of the previous studies of the interaction of metal cations and quinolones. This study is the first to examine the possibility of interaction in the blood circulation using ciprofloxacin and ferrous sulfate as representative in the dogs model, and identify changes, if any, in the pharmaceutical antibiotics. To limit the direct physical interaction in the gastrointestinal. the current study design required the dogs (2.5 –3.5 Kg) to be dosed with 100 mg/kg of oral ferrous sulfate and 10 mg/kg of intravenous ciprofloxacin. Control animals received only intravenous ciprofloxacin. Blood samples were collected over time for quantitation of ciprofloxacin independently by HPLC assays. Results showed that the disposition of ciprofloxacin in control animals was biexponential with a (mean±SE) terminal elimination half-life (5.33 ± 0.14),(7.70 ± 0.08) respectively. The volume of distribution (Vd, 1.10± 0.15 L/kg), (Vd, 1.68 ± 0.33L/kg) was observed. When the antibiotic was dosed with oral iron, appeared to show a wider distribution and a longer elimination of ciprofloxacin relative to controls, antibiotic exposure (AUC 0-∞ ) was also significantly higher in the presence of iron (65.43 ± 1.60, 67.95 ± 1.78mg/ml/hr.). In conclusion, concomitant dosing oral iron lead to significant changes in the pharmacokinetics of intravenous and oral ciprofloxacin.
Keywords | Ciprofloxacin, Quinolones, Ferrous sulfate, Iron interaction, Metal cations, Pharmacokinetics.
Editor | Kuldeep Dhama, Indian Veterinary Research Institute, Uttar Pradesh, India.
Received | December 11, 2016; Accepted | January 26, 2017; Published | February 25, 2017
*Correspondence | Orooba Mohammed Saeed Ibrahim, Department of Physiology and Pharmacology, College of Veterinary Medicine, Baghdad University, Iraq; Email: oroobam2000@gmail.com
Citation | Ibrahim OMS, Shakir AF (2017). Pharmacokinetic Interactions Between Intravenous Ciprofloxacin and Oral Ferrous Sulfate. Adv. Anim. Vet. Sci. 5(2): 70-77.
DOI | http://dx.doi.org/10.14737/journal.aavs/2017/5.2.70.77
ISSN (Online) | 2307-8316; ISSN (Print) | 2309-3331
Copyright © 2017 Ibrahim and Shakir. This is an open access article distributed under the Creative Commons Attribution License, which permits unrestricted use, distribution, and reproduction in any medium, provided the original work is properly cited.
INTRODUCTION
Fluoroquinolones, synthetic broad spectrum bactericidal agents, are gaining wide spread acceptance in veterinary medicine to treat urinary tract, respiratory tract, gastrointestinal tract, skin and soft tissue infections (Khan et al., 2011). Ciprofloxacin, 1-cyclopropyl-6 fluoro-1, 4-dihydro-4-oxo-7-(1piperazinyl)-3 quinolone carboxylic acid, Showed better antimicrobial activity than the parents compounds nalidixic acid. Second-generation ciprofloxacin show greater potency, lower toxicity and broader antibacterial spectrum. The main difference between ciprofloxacin and other antibiotics is that it can be administered parentally, topically and orally. It is well absorbed and widely distributed into various body tissues and fluids (Andersson and MacGowan, 2003; Khan et al., 2011).
Iron is an essential component of every cell in the body. Although known for its crucial role in the transport and storage of oxygen in the hemoglobin and myoglobin, respectively, among a great variety of iron group of enzymes also act as a carrier of electrons a catalyst for oxygen, hydroxyl, and is essential for cell growth and proliferation. Iron supplements administered on a large scale for the treatment of anemia due to iron deficiency, especially in chronic diseases such as kidney disease (Gotloib et al., 2006), heart failure (Nanas et al., 2006), or inflammatory bowel disease (Gasche et al., 2004). Without an adequate supply of iron, hemoglobin cannot be synthesized and the number of red blood cells in the blood cannot maintain a sufficient Level (Kalantar-Zadeh et al., 2009).
Iron is available widely in dietary supplements also, its ability to interfere with the absorption of oral ciprofloxacin (Kara et al., 1991; Letho et al., 1994). In humans, concurrent oral administration of 500 mg of ciprofloxacin with 100 mg of ferrous sulfate significant reductions in the area under concentration vs. time curve, peak plasma concentration and urinary recovery of antibiotics as much as 57%, 54% and 58%, the respectively.
Therefore, it is recommended that an adequate dosing interval should be spaced between the two agents. Interestingly, the presence of iron also cause slower elimination of antibiotics and it was evident in the pharmacokinetic profiles presented (Letho et al.,1994); however, this observation has not addressed by the authors. There are a number of recent studies in laboratory also show, in addition to lower oral bioavailability, slower elimination and wider distribution of ciprofloxacin when it is orally administered with a number of mineral-rich herbs (Zhu et al.,1999 a; Zhu et al.,1999 b). Pharmacological perspective, this interaction not only impacts on oral bioavailability, but the disposition antibiotic properties. The fact taken the metal complex also reduces the antimicrobial activity in the laboratory, and the possibility that pharmaceutical parallel rotation, and quinolones cannot be ruled out (Papich, 2012). To test this hypothesis, ciprofloxacin and iron were employed as representative interactants representational.
The purposes of this investigation were to study the effect of ferrous sulphate on the absorption of ciprofloxacin and to determine whether probable interactions could be prevented. And to make sure the interaction between ciprofloxacin and iron is not only confined to the gastrointestinal tract but also occurs in the systemic circulation. Therefore, this experiment aimed to study is to evaluate the Pharmacokinetics of single dose Ciprofloxacin after intravenous injection in healthy dogs and Pharmacokinetics interaction of co-administration ciprofloxacin and ferrous sulphate at different time and study the effect of ferrous sulphate on the bioavailability of ciprofloxacin.
MATERIALS AND METHODS
Chemical reagents and apparatus
Ciprofloxacin was kindly provided as a gift by VAPCO Company. Internal standard was employed for the ciprofloxacin assay by HPLC and was purchased from (VAPCO company). Ferrous sulfate, acetonitrile (HPLC grade), triethylamine and other chemical reagents were acquired commercially. HPLC equipped with UV-visible detector series 200. The analyte were separated using Hypersil C18 Column. (250 x 4.6 mm, 4.5 μ-particle sizes).The UV-visible detector adjusted at 280 nm.
Pharmacokinetic Studies
Twenty Male dogs (2.5-3.5 Kg) were housed under controlled conditions. The animals were kept in individual cages, fed and water ad libitum. They were submitted to experimentation after a 14-day adaptation period. A cannula was surgically inserted into the right jugular vein of each study animal one day prior to blood sampling. These animals were fasted overnight before drug dosing on the next day. In the test group (n=10), dogs were given an oral 100mg/kg dose of ferrous sulfate which was followed immediately by an i.v. bolus dose of ciprofloxacin at 10 mg/kg. Dogs receiving only the same i.v. dose (10 mg/kg) of ciprofloxacin were used as controls (n=10). The aqueous drug solutions were freshly prepared prior to dosing. As there was no prior knowledge of the existence of a systemic interaction between quinolones and metal cations, the selection of doses and dosing strategy for the two interactants was aimed at maximizing the interaction and avoiding any interference in data interpretation by reduced oral absorption. Blood was withdrawn via the cannula just prior to ciprofloxacin administration (t=0) and at 0.08, 0.16, 0.25, 0.33, 0.5, 0.75, 1, 1.5, 2, 3, 4, 6, 8, and 12 hr. after dosing. From these samples, serum was immediately separated by centrifugation at 10,000 g for 5 min and was divided into two 100 mL aliquots. Serum sample were stored at -80oC until subsequent ciprofloxacin analyses by both HPLC assays.
Preparation of Mobile Phase
Acetonitrile and 0.25 M H3PO4 (60:40 v/v) were used as mobile phase (pH=3.0) and pumped at a rate of 1.5 ml.min-1. The UV-visible detector adjusted at 280 nm.
Calibration Curve
Solution (100μg/ml) of the ciprofloxacin, used as internal standard, was prepared in mobile phase and the diluted accordingly with the same solvent system. Calibration curve was constructed in the range of 5 ng to 50 ng/ml in mobile phase and 10 ng to 80 ng/ml in dog serum.
Sample preparation
Serum samples were precipitated for measure by HPLC that made by take 200 µL of serum sample with 600 µL of acetonitrile in 2 ml Eppendroff tube and centrifuged at 10,000 rpm for 10 minute. The clear supernatant was collected and evaporated at 45 0C under flux of nitrogen. The residue was then reconstituted in mobile phase (100 μL) and 20-μL sample was injected into HPLC (Khan et al., 2011).
Limit of detection and limits of quantifications
The method was validated for recovery, accuracy, precision, limit of quantification (LOQ), limit of detection (LOD), linearity, and selectivity. The % recovery in serum was measured by comparing peak area of ciprofloxacin in sample with the peak area in sample from direct injection of an aqueous solution containing the same amount of drug (n =7). LOD was expressed as a concentration for which the signal-to- noise ratio (S/N) was equal to 3. LOQ of the assay was evaluated as the concentration for which S/N was equal to 10. The selectivity of the method was described as the resolution of the ciprofloxacin peak from other peaks in the matrics (Samanidou et al., 2004). Calibration curve was obtained by plotting peak area ratio of ciprofloxacin and internal standard against ciprofloxacin concentration.
Estimation of pharmacokinetic parameters for ciprofloxacin
Maximum concentration in serum and the time required to reach this concentration were obtained from the observed data for each dogs. The area under the serum concentration-versus-time curve was calculated by using a linear trapezoidal estimation. The serum concentration-time data of ciprofloxacin were assessed by compartmental analysis. Least square regression analysis was employed directly on the data in both the distribution and terminal elimination phases for the estimation of apparent distribution (α) and elimination (β) rate constants, respectively.
RESULTS
The retention times for ciprofloxacin was5 min. The blank serum was used to establish the base line and then serum samples were spiked with the ciprofloxacin to study any interference peaks from the biological matrices. The results showed no any interference from endogenous components.
Linearity and accuracy
The linearity of the calibration curves was verified from 5 µg to 50 µg/ml for ciprofloxacin in mobile phase and 10 µg to 80 µg/ml in dog serum. The linear curve was observed in the concentration range of the respective samples. And the r2 value calibration curve in mobile phase and serum samples was 0.996, 0.998, respectively. The regression equations show good intercept and slopes values (Figure 1 and 2).
Limit of detection (lod) and limit of quantification (loq).
The limit of quantitation of the assay was evaluated as the concentration equal to ten times the value of the single-to-noise ratio. This was taken as the lowest concentration in the calibration range. The limit of detection (LOD) for serum is 1 ng.ml-1 while limit of quantitation (LOQ) for dog serum was 3.33 ng.ml-1.
Single intravenous injection of ciprofloxacin
Following intravenous administration of the study antibiotic to the control animals, serum ciprofloxacin concentrations (mean ± SE), time curve following a single intravenous of 10 mg/kg body weight in dogs are shown in (Figure 3). Mean serum concentration of the drug was found to be (33.01 ± 2.90 µg/ml) at 5 min which rapidly declined to (13.06 ± 1.34 µg/ml) at 45 min and after that reduced gradually to (4.21 ± 0.78 µg/ml) at 6 hours . The serum ciprofloxacin concentration versus time data was best fitted to a biexponential equation corresponding to a two compartmental open model. The serum concentrations of ciprofloxacin declined in a biexponential manner showing a rapid distributive phase and as lower elimination phase (3.46 ± 0.13, 0.13 ± 0.01hr-1) respectively. From the data generated using the HPLC assay, rapid distribution of the antibiotic was clearly evidenced by the short distribution half-life ( t 1/2 α: 0.20 ± 0.05 h-1) and the 2-fold reduction in the mean serum from ( 33.01 ± 2.90 µg /ml) to (17±1.24 µg/ml) over the first 30 minute following drug administration. Furthermore, large apparent volume of distribution (Vd 1.10 ± 0.15 L/kg) clearly approved the rapid distribution of Ciprofloxacin in this experiment. The compartmental pharmacokinetic parameters (mean ± SE) after intravenous administration of the ciprofloxacin in dogs are presented in (Table 1), (Figure 4). Following compartmental model, the distribution and elimination half-life of ciprofloxacin in dogs was found to be (0.20 ± 0.05, 5.33 ± 0.14 hr-1) respectively.

Figure 3: Serum ciprofloxacin concentrations (mean ± se) in 5 dogs after intravenous injection at dose (10 mg / kg bw).
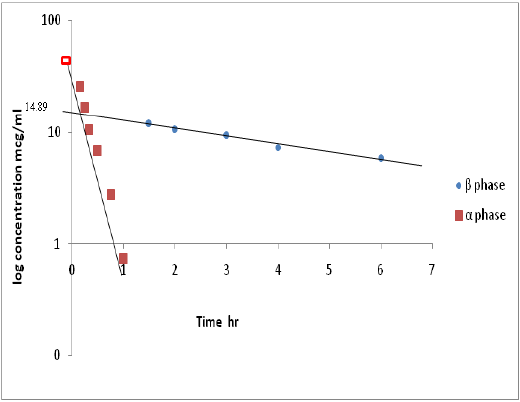
Figure 4: Semi-log plot of µg/ml versus time (hr) shows residual line and µg/ml late line after iv injection of ciprofloxacin.
The Volume of distribution V.d , Area under the curve AUC , and Clearance CL , values were calculated to be (1.10 ± 0.15 L/Kg), (65.43 ± 1.60 mg/ml.hr) and (0.15 ± 0.04 L/kg/hr), respectively. Further, the values of K12, K21 and the ratio of the drug concentration between the tissue and central compartment (T/C) were (2.27 ± 0.18),( 0.75 ±0.05 ) and ( 3.01 ) respectively.
Table 1: Pharmacokinetic parameters of Ciprofloxacin after Intravenous injection (Mean ± SE).
Pharmacokinetics parameters |
Mean ± SE |
Distribution rate constant (α) hr-1 |
3.46 ± 0.13 |
Elimination rate constant (β) hr-1 |
0.13 ± 0.01 |
Serum concentration at α phase at time zero (A) (µg /ml) |
31.60 ± 0.27 |
Serum concentration at β phase at time zero (B) (µg/ml) |
7.32 ± 0.45 |
t 1/2 α hr |
0.20 ± 0.0 5 |
t 1/2 β hr |
5.33 ± 0.14 |
Volume of distribution Vd (L/kg) |
1.10 ± 0.15 |
Area under curve AUC (mg/ml.hr) |
65.43 ± 1.60 |
Clearance CL (L/kg/hr) |
0.15 ± 0.04 |
K12 |
2.26 ± 0.15 |
K21 |
0.75 ± 0.02 |
Single IV injection of Ciprofloxacin and Single oral administration of Ferrous Sulphate given simultaneously
Pharmacokinetic analyses of the concentration vs. time data generated by the HPLC revealed significant differences in the parameter estimates between the two groups (A and B). In particular, the extent of average drug exposure as reflected by AUC0-∞ significantly (P < 0.05) increase from (65.43 ± 1.60 mg/ml.hr) in control animals to (67.95 ± 1.78 mg/ml.hr) in dogs dosed with ferrous sulfate that given at same time. Such an increase in exposure mirrored the significant decrease in clearance CL observed (0.15 ± 0.04vs. 0.14 ± 0.02L/kg/h), (Table 2). Furthermore, volume of distribution was significant (p<0.05) in animals receiving oral ferrous sulfate (1.10 ± 0.15 vs.1.68 ± 0.33 L/kg). Also terminal elimination rate constant of the ciprofloxacin also markedly increase significant (p<0.05) (5.33 ± 0.14, 7.70 ± 0.08 h -1), while Elimination rate constant (β) decreased from (0.13 ± 0.01 to 0.09 ± 0.01 h-1).
Although significant changes in the overall pharmacokinetic system were observed, t1/2α was not significantly altered. Pharmacokinetic parameter estimates obtained for ciprofloxacin in dogs receiving ferrous sulfate co administration are provided in (Table 2) and (Figure 5 and 6).
Table 2: Pharmacokinetic parameters of Ciprofloxacin after Intravenous injection when given simultaneously with ferrous sulfate administrated orally (Mean ± SE).
Pharmacokinetics parameters |
Mean ± SE |
Distribution rate constant (α) hr-1 |
1.38 ± 0.28 |
Elimination rate constant (β) hr-1 |
0.09 ± 0.01 |
Serum concentration at α phase at time zero (A) (µg /ml) |
24.45 ± 1.45 |
Serum concentration at β phase at time zero (B) (µg /ml) |
4.91 ± 0.37 |
t 1/2 α hr |
0.37 ± 0.02 |
t 1/2 β hr |
7.70 ± 0.08 |
Volume of distribution Vd (L/kg) |
1.68 ± 0.33 |
Area under curve AUC (mg/ml.hr) |
67.95 ± 1.78 |
Clearance CL (L/kg/hr) |
0.14 ± 0.02 |
K12 |
1.1 ± 0.19 |
K21 |
0.37 ± 0.04 |

Figure 5: Serum ciprofloxacin concentrations (mean ± se) in 5 dogs after intravenous injection at dose (10 mg /kg b.w) with oral ferrous sulfate (100mg/ kg) given at same time
DISCUSSION
The decision as to which model best fits the serum concen-trations of ciprofloxacin was based, after calculation of 10 mg/kg body weight oral and intravenous the median values, on both the visual comparison of the regression curve with the measured serum concentrations and on the comparison of the relative squared residuals. The results were confirmed by evaluating the individual data.
According to these criteria, after intravenous injection of ciprofloxacin 10 mg and oral administration, the serum time curve could be the best as described by an open two compartment model. Furthermore, the goodness of fit

Figure 6: Semi-log plot of µg/ml versus time (hr) shows residual line and µg/ml late line after iv injection of ciprofloxacin with ferrous sulphate.
and the comparison of the residuals were used for the decision not to use the 24-hr values of the serum concentration this phenomenon was reviewed by (Hoffren et al., 1985).after intravenous administration, serum ciprofloxacin concentration (mean± SE) versus time data in dogs were best fitted to a two-compartment open model. This is comparable with the results of other studies in chicken (Anadoan et al., 2001), and in rabbits (Sahar and Eman, 2014), sheep (Munoz et al., 1996), goats (Raina et al., 1994), pre-ruminant calves (Nouws et al., 1988) and dogs (Abadia et al., 1995). Based on the compartmental model of analysis, distribution kinetic parameters, namely-distribution rate constant (α;3.46 ± 0.13 h-1), distribution half life (t1/2 α; 0.20 ± 0. 05hr-1) and rate constants of the transfer of drug from central to peripheral compartment K12 (2.26 ± 0.15h-1) and peripheral to central K21 (0.75 ± 0.02 h-1) suggested that rapid distribution of the drug from blood to tissues and body fluids.
In current study Distribution half-life of ciprofloxacin in dogs (0.20 ± 0.0 5) hours was longer than that in sheep (2.38 min) (Munoz et al., 1996), and pigs and pre-ruminant calves (Nouws et al., 1988), and in calves (7.8 min) suggesting thereby that the disposition kinetic behavior of drugs differ among different species of animals.
The ratio of transfer rate constants of ciprofloxacin from central to peripheral compartment and vice versa (K12/K21; 3.01) suggested that this drug possess much tendency to accumulate in body tissues; and thus less likely to adversely affect the human or animal health due to drug residues. Further, the apparent volume of distribution (Vd) (1.10 ± 0.15 L/kg) suggests substantial tissue penetration ability of the drug. The Vd value of ciprofloxacin in dogs was markedly lower as compared to the corresponding value of 2.50 L/kg in pre-ruminant calvesalso comparable to that of 1.89L/kg in sheep and 1.71+0.09 L/kg in calve (Mohan and Garg, 2003).
The High AUC value of (65.43 ± 1.60 mg/ml.h), of ciprofloxacin in this study also adds credence to the fact that this antibacterial agent distributes widely in body fluids and tissues of dogs and hence could be effectively used for the treatment of various systemic as well as deep seated infections and this is almost agree with work of (McKellar, 1996).
The elimination half-life (t1/2b) of ciprofloxacin in dogs (5.33 ± 0.14hr-1). was greater comparable to that of 2.69 h and 2.44 h in calves and in pre-ruminant calves and 1.2h-1 in sheep respectively (Munoz et al., 1996), was almost comparable that of 4.85 h in horses (Yun et al., 1994), suggested that species-dependent differences in pharmaco-disposition of ciprofloxacin.
The current study were comparable to studies by (Walker et al., 2000), in which ciprofloxacin administered at dose of 10 mg/kg yield a Cmax of 12.9 ± 1.43 µg/ml after oral administration in dogs. The oral bioavailability of ciprofloxacin was 83.98 % in this study, which was higher than in chicken 69.12 % (Anadoan, 2001) and 53.0 % reported in calves and 37.3 % in pigs of another study (Nouws et al., 1988). The relatively low bioavailability of ciprofloxacin in calves and pigs may be caused by biliary excretion or extensive metabolism of the drug.
In mammal species, the metabolism of ciprofloxacin is still not fully elucidated although the piperazinyl ring seems to be the centre of metabolism; the ring may become oxidised, opened, formylated or sulphated. However, oxociprofloxacin and desethyleneciprofloxacin were minor metabolites in calves, and these metabolites were absent in pigs (Nouws et al., 1988). Presumably other, more important, metabolic pathway(s) for ciprofloxacin may be present in calves and pigs, or extensive degradation/conversion of ciprofloxacin may occur in the gastrointestinal tract. In this context, species and feeding are of importance in the extent of metabolism, bioavailability and drug biodegradation in the gastrointestinal tract following oral ciprofloxacin administration.
Fluoroquinolones exert concentration-dependent bactericidal effect. To maximize their clinical efficacy, it is important to obtain plasma Cmax to MIC ratio (Cmax/MIC) of 8-12 (Mouton et al., 2002). Bacterial re growth due to resistant-sub-populations can be prevented when peak concentration of fluoroquinolones exceeds the MIC values of pathogens by a factor of 8 or more (Boothe et al., 2006).
Therefore, dosage regimen of fluoroquinolones should be computed to achieve the maximal bactericidal effect. Considering the MIC value of 0.5 µg/ml against most of the susceptible pathogens, the Cmax:MIC ratio of ciprofloxacin in dogs in the present study was found to be 11.42 which suggests that a dose of 5 mg/kg is sufficient to provide therapeutically satisfactory concentrations for almost 8hr. However, due to concentration-dependent killing effect, its post antibiotic effect and the pharmacodynamics-pharmacokinetic relationship (Cmax/MIC) value greater than 8, ciprofloxacin may be administered to dogs at 24 hours interval by intravenous route. For more severe infections requiring higher plasma concentrations (>1.0 µg/ml), ciprofloxacin may be administered at a loading dose of 4.5 mg/kg and subsequently maintained at 3.2 mg/kg repeated at 8 hourly interval.
Chelate formation by metal cations is a known problem for ciprofloxacin and other quinolone antibiotics (Darcy and McElnay, 1985). Indeed, such interactions can cause problems in two distinct directions for antibiotics: reduction in oral bioavailability and decrease in antimicrobial activity. Although the two phenomena can in theory, co-exist, most previous interaction studies have focused only on the extent of reduction in oral bioavailability. The possible influence of metal cations on the antimicrobial activity of quinolones present in the systemic circulation, however, has not been explored.
The significant alterations in the pharmacokinetic behavior of ciprofloxacin observed in this study suggest the presence of a drug-drug interaction between the antibiotic and iron even though two independent dosing routes were employed. With concomitant oral ferrous sulfate, systemic ciprofloxacin exposure (AUC0→∞) assessed by the HPLC was 50% higher than that of the control and reduction observed in the clearance in group (B) that given ciprofloxacin with ferrous sulphate stumenoasuly this result agreement with (Wong et al., 2013).
In addition, the 2-1 fold increase in t 1/2 β and volume of distribution as a result of the oral iron dosing also contributed to the slower drug clearances. Although this slower elimination is also apparent from data obtained in previous human and animal studies (Letho, 1994; Zhu, 1999 a,b). The higher propensity for tissue distribution, slower elimination and lower renal clearance suggest the formation of amore lipophilic metal chelate. Since extensive tissue distribution and high cellular uptake of ciprofloxacin are important attributes for its activity against intracellular infections, it has to be kept in perspective that wider distribution of the presumably inactive chelates in the presence of iron may actually attenuate this important effect of the antibiotic (Geisser and Muller, 1987; Anderson and Osheroff, 2001; Pommier et al., 2010).
In the presence of iron, ciprofloxacin tended to stay longer in the body; this, however, was not matched by the anticipated increase in antimicrobial activity due to lower concentration of ciprofloxacin especially after oral ferrous sulfate administration where scored lower concentration than Cmax to MIC ratio, this result approve by (Wong et al., 2013), whose found plasma ciprofloxacin concentrations as assessed by the agar diffusion assay, were apparently lower than those of the HPLC assay. This reduction in antimicrobial activity strongly indicates a negative impact of iron on the antibiotic’s activity on formation of the metal chelates. Interestingly, the reduced activity in plasma was more noticeable after oral ferrous sulfate administration. The time frame of shift in activity observed approximates the absorption profile of iron reported in a previous study in which maximum plasma iron concentration is achieved at approximately 2 hour in rats after administration of oral ferrous sulfate (Geisser and Burckhardt, 2011). Therefore, the impact of iron on the pharmacokinetics of ciprofloxacin was more apparent during terminal elimination of the antibiotic, when the potential for formation of chelates in the systemic circulation was highest.
These findings showed that the interaction between ciprofloxacin and ferrous sulfate is more complicated than a sole physical interaction. In particular, the presence of antibiotic in the systemic circulation as determined by the HPLC assay does not guarantee a proportional degree of antimicrobial activity.
Under such circumstances, interpretation of concentration is dependent on the type of assay employed and the conclusions drawn can be misleading if existence of such an interaction is not known or is ignored. In other words, the amount of ciprofloxacin in serum determined chemically may not be equivalent to that measured microbiologically when in the presence of cations. This was confirmed by (Schmitz et al., 2002; Mouton et al., 2005; Zhou et al., 2008).
ACKNOWLEDGEMENT
Authors acknowledge to the Department of Physiology and Pharmacology, College of Veterinary Medicine, Baghdad University, Baghdad, Iraq.
CONFLICTOF INTERESTS
There exists no conflict of interest.
AUTHORS’ CONTRIBUTION
All the authors have contributed equally in terms of giving their technical knowledge to frame the article.
REFERENCES