Advances in Animal and Veterinary Sciences
Research Article
The Impact of Cinnamon Oil on Hepatorenal Toxicity and Antioxidant Related Gene Expression Induced by Deltamethrin in Rat
Walaa M.S. Ahmed1, Naglaa M. Abdel-Azeem2, Marwa A. Ibrahim3*, Nermeen A. Helmy4, Abeer M. Radi5
1Department of Clinical Pathology, Faculty of Veterinary Medicine, Beni-Suef University, Beni-Suef, 62511, Egypt; 2Department of Animal and Poultry Management and Wealth Development, Faculty of Veterinary Medicine, Beni-Suef University, Beni-Suef, 62511, Egypt; 3Department of Biochemistry, Faculty of Veterinary Medicine, Cairo University, Giza Egypt; 4Department of Physiology, Faculty of Veterinary Medicine, Beni-Suef University, Beni-Suef, 62511, Egypt; 5Department of Pharmacology, Faculty of Veterinary Medicine, Beni-Suef University, Beni-Suef, 62511, Egypt.
Abstract | The protective role of cinnamon oil (C. oil) on the liver and renal functions, Nrf2 gene expression and histopathology in the rat following administration of deltamethrin (DM) was investigated. Rats were divided into 4 groups, rats of the 1st group (control) were given saline, while the 2nd group was given C. oil (100 mg/kg B.W) and 3rd group was administrated DM (6 mg/kg B.W) orally. The 4th group was given C. oil and DM. All treatments were given orally daily for 3 weeks. Blood biochemical parameters and histopathological study for liver and kidney tissues were conducted. Administration of DM caused a significant elevation in alanine aminotransferase, aspartate aminotransferase and alkaline phosphatase activities, cholesterol, triglyceride, urea and creatinine concentrations and upregulates the Nrf-2 gene expression. While the total protein, albumin and globulin levels were not changed. It was noticed that concomitant oral administration of C. oil plus DM significantly decreases the activities of these enzymes, cholesterol, triglyceride, urea and creatinine concentrations. Also, C. oil resulted in down-regulation of Nrf-2 expression and ameliorated the histopathological alterations in hepatic and renal tissues. In conclusion, Co-administration of C. oil attenuates the hepato-nephrotoxic effects of DM and this may be through decreasing oxidative stress.
Keywords | Cinnamon oil, Deltamethrin, Kidney, Liver, Nrf-2 gene, Histopathology.
Received | February 06, 2021; Accepted | March 17, 2021; Published | June 15, 2021
*Correspondence | Marwa A Ibrahim, Department of Biochemistry, Faculty of Veterinary Medicine, Cairo University, Giza Egypt; Email: Marwa199@yahoo.com
Citation | Ahmed WMS, Abdel-Azeem NM, Ibrahim MA, Helmy NA, Radi AM (2021). The impact of cinnamon oil on hepatorenal toxicity and antioxidant related gene expression induced by deltamethrin in rat. Adv. Anim. Vet. Sci. 9(7): 1071-1077.
DOI | http://dx.doi.org/10.17582/journal.aavs/2021/9.7.1071.1077
ISSN (Online) | 2307-8316; ISSN (Print) | 2309-3331
Copyright © 2021 Ibrahim et al. This is an open access article distributed under the Creative Commons Attribution License, which permits unrestricted use, distribution, and reproduction in any medium, provided the original work is properly cited.
Introduction
Due to the widespread and intensive use of deltamethrin (DM) in agriculture and public health, it has become one of the most environmental and industrial pollutants that are toxic to humans, animals, fishes and birds. The most common sources of human and animal exposure to deltamethrin are polluted water and food (Kumar et al., 2015). The liver and kidney are reported among the most common tissues that are affected due to DM exposure since the liver is the main site of DM metabolism and the kidney is the principal organ of its elimination (Abdel-Daim et al., 2013). Deltamethrin is oxidized by cytochrome P450 enzymes in the liver and elevates the reactive oxygen species (ROS) level (Rehman et al., 2017). Critical events in the toxicity of DM are the induction of apoptosis in various cells (Kumar et al., 2015) and oxidative stress which can further cause mitochondrial damage and lead to liver injury and nephrotoxicity (Maalej et al., 2017).
Cinnamon oil (C. oil) is obtained from the Cinnamomum species and has been used in the pharmaceutical and food industries for its medicinal and nutritional properties.
Volatile oil isolated from different parts of cinnamon have the same form of monoterpene hydrocarbons in different ratios but with a varied distinctive constituent e.g. presence of cinnamaldehyde, eugenol and camphor in the leaf oil and root-bark oil, bark oil respectively (Kaur and Shri, 2018).
Recently, many investigators have shown that Cinnamomum species have been capable to suppress oxidative stress and decrease lipid peroxidation (Jain et al., 2015; Abdelwahab et al., 2017).
Therefore, this study attempted to explore the effect of cinnamon oil on hepato-nephrotoxicity induced by the oxidative stress caused by deltamethrin in rats.
Materials and Methods
Chemicals
Deltamethrin (DM) was purchased from the Arab company for Chemical ind., Egypt. Cinnamon oil was obtained from El-Captain Company, for extracting natural oils, El-Obour CityEgypt. Kits were purchased from Biodiagnostic company, Chemical Co., Egypt.
Animals and Experimental design
Male Wistar albino rats (100-200 g) were purchased from the animal house of El Nahda University. The rats had free access to food and tap water ad libitum and were allowed to acclimatize for one week. This study was performed following the Institutional Animal Care and Use Committee of Beni-Suef University (BSU-IACUC) ethical guidelines. Forty rats were randomly assigned to 4 groups. Group (1) rats were received physiological saline, group (2) orally received C. oil at a dose of 100 mg/kg B.W., group (3) orally received DM alone at a dose of 6 mg/kg B.W. (Sharma et al., 2014), and group (4) orally administrated with C. oil and DM at previously mentioned doses daily. After three weeks of administration, rats were anesthetized with 5% diethyl ether and blood samples from all groups were collected from the retro-orbital plexus then rats were sacrificed with cervical dislocations. Blood samples were centrifuged to get serum for biochemical examination. Livers and kidneys of rats were quickly removed and washed and some of which were fixed in 10% formalin solution for histopathology, while other parts of the liver and kidney tissue samples were stored at -80°C for NF-E2-related factor 2 (Nrf-2) gene analysis.
Assessment of Biochemical Parameters
Liver function test: Serum liver enzymes activities of alanine aminotransferase (ALT) and aspartate aminotransferase (AST) and alkaline phosphatase (ALP), total proteins, albumin, cholesterol and triglycerides were measured colormetrically according to the manufacturer’s instructions. The globulin concentration was estimated by subtracting the albumin value from the total protein value for obtaining the albumin-globulin (A/G ratio).
Estimation of urea and creatinine: Urea was estimated as described by Patton and Crouch (1977). Creatinine was determined by buffered kinetic Jaffe’s reaction method as described by Bowers and Wong (1980).
RNA isolation and real-time PCR for Nrf-2 gene expression: The RNeasy mini kit (Qiagen) was used to isolate the total RNA from the samples according to the manufacturer’s instructions. The purity and concentrations of the extracted t-RNA were measured spectrophotometrically. The RevertAid First Strand cDNA Synthesis (Thermo scientific #K1622) was used to generate the First-strand cDNA (Yassin et al., 2016). The primer sets used to amplify Nrf-3 were designed using Primer3 software were sense primer: 5’-GGCCCTCAATAGTGCTCAG-3’; antisense primer: 5’-TAGGCACCTGTGGCAGATTC-3’. The quantitative real-time PCR was done using a Real-Time PCR System (Applied Biosystems, USA) for 40 cycles of denaturation at 95°C for 30 s, annealing at 58°C and extension at 72°C for 30 s. The GAPDH gene was used as the internal standard to normalize the expression values (Ibrahim et al., 2020). Each sample was assessed in triplicates. The CT values were used to calculate the gene/ GAPDH ratio, with a value of 1.0 used as the control (calibrator) (Morgan et al., 2017). The normalized expression ratio was calculated using the Mxpro software (Khalaf et al., 2019).
Histopathological Evaluation
Specimens (liver and kidney) from all rat groups were performed according to the method of Banchroft and Gamble (2008). After fixation with 10 % neutral buffered formalin, specimens were washed in water, dehydrated in ascending grades of alcohol, then in xylene. Then, samples were embedded in paraffin for preparation of 5 µm paraffin sections and then stained with hematoxylin and eosin.
Statistical Analysis
One-way analysis of variance (one-way ANOVA) was used to elucidate significant differences between different groups. Tukey’s post hoc test was used for multiple comparisons. All statistical analyses were done using GraphPad Prism version 5.0 software. The criterion of statistical significance was considered statistically significant at <0.05. Results were represented as Mean ± SE.
Table 1: Values of biochemical parameters of different groups.
Parameters | Control | C. oil | DM | C. oil +DM |
ALT (U/L) | 18.0±0.7 | 18.6±0.8 |
31.2±1.40a,b |
21.9±0.74c |
AST (U/L) | 19.8±1.4 | 17.7±1.05 |
28.1±2.24a,b |
23±1.16 |
ALP (U/L) | 76.1±2.7 | 75.2±6.5 |
133±4.9a,b |
91.3±4.1c |
Total protein (g/dl) | 6.9±0.31 | 6.7±0.52 | 5.89±0.23 | 6.1±0.21 |
Albumin (g/dl) | 2.8±0.17 | 3.1±0.08 | 2.87±0.15 | 2.4±0.28 |
Globulin (g/dl) | 4.1±0.36 | 3.6±0.59 | 3.13±0.12 | 3.7±0.25 |
A/G ratio | 0.6±0.08 | 1.0±0.28 | 0.98±0.06 | 0.70±0.11 |
Cholesterol (mg/dl) | 53.2±1.9 | 56.1±3.0 |
100±3.9a,b |
63.00±1.8c |
Triglycerides (mg/dl) | 36.0±0.9 | 34.0±1.6 |
64.3±3.4a,b |
43.5±2.4c |
Urea (mg/dl) | 40.0±1.48 | 42.7±1.54 |
62.61±2.14a,b |
49.3±2.80 |
Creatinine (mg/dl) | 1.89±0.29 | 1.00±0.19 |
8.11±0.73a,b |
1.4±0.40c |
Data are expressed as means ± SE with dissimilar superscript letters (significantly differing at P < 0.05): a) significantly different from control value; b) significantly different from C. oil group; c) significantly different from theDM group.C. oil, represents Cinnamon oil; DM represents deltamethrin and C. oil+DM, represents cinnamon oil and deltamethrin.
Results
Blood biochemical parameters
To characterize the liver function in response to DLM exposure, we measured the activities of serum AST, ALT and ALP in different groups. There were no significant changes in the enzyme activities between C. oil-treated rats from the control group (Table 1). The present data showed that in the DM group, significant increases of AST, ALT and ALP activities were detected as compared with control and C. oil groups. The enzymes’ activities were significantly declined in the C. oil plus DM group as compared to the deltamethrin alone group. On the contrary, co-administration of rats with C. oil and DM prevented a DM-induced increase in the enzyme activities when compared to control and C. oil groups. From Table (1), we observed that the DM did not affect the total protein, albumin, and globulin concentrations as compared to the control group.
Moreover, the cholesterol level found to be increased in the DM group comparing to the control. While in the C. oil plus DM group, the total cholesterol and triglycerides concentration was significantly decreased as compared to the control group. The concentration of cholesterol and triglycerides were significantly decreased in the group of rats administrated C. oil plus DM as compared to the DM alone group.
In the deltamethrin group, there was a significant increase in levels of urea and creatinine were observed as compared to the control and C. oil groups. A significant decrease in the concentration of creatinine was observed in the C. oil plus DM group compared to the control and C. oil groups. On the other hand, C. oil had no significant effects on urea and creatinine compared to the control group.
Nrf-2 gene expression
The transcript levels of Nrf-2 (Figure 1) showed significant up-regulation in the DM treated group relative to the control group. Co-treatment of cinnamon and deltamethrin showed an improving effect such that the mRNA levels were decreased if compared to the intoxicated rats.

Figure 1: Real time PCR for Nrf-2 gene expression in liver and kidney of different experimental groups.
Data are expressed as means ± SE with dissimilar superscript letters (significantly differing at P < 0.05): a) significantly different from control value; b) significantly different from C. oil group; c) significantly different from the DM group. C. oil, represents Cinnamon oil; DM represents deltamethrin and C. oil+DM, represents cinnamon oil and deltamethrin
Histopathological results
Microscopic results showed that liver sections of the control (Fig. 2a) and C. oil (Fig. 2b) groups appeared normal. While liver of the DM group exhibited marked portal vein congestion, diffuse hydropic degeneration, necrosis with inflammatory cell infiltrations (Fig. 2 d, e). Regeneration of hepatocytes and improvement in central vein and sinusoidal blood was observed in the C. oil plus DM treated group (Fig. 2f).

Figure 2: a. Liver tissue of control showing normal cell plates formed of polygonal hepatocytes with granular eosinophilic cytoplasm and vesicular nuclei & intervening regular sinusoids and normal central veins (black arrows). (H&E stain ×100). b. Liver of C. oil treated rat showing normal structure of the liver with normal central vein, sinusoids and hepatocytes (H&E stain ×100). c. Liver of DM treated rats showing marked portal veins congestion (black arrow) associated with widely diffuse hydropic degeneration of hepatocyte (H&E stain ×100). d. Liver from DM group showing diffuse marked hepatocellular hydropic degeneration with dilated hepatic sinusoids and multiple foci of hemorrhage (black arrows) with areas of necrosis showing ghost of hepatocytes some of hepatocytes revealed condensed/pyknotic nuclei (red circle) with aggregates of mononuclear inflammatory cells (blue arrows) ).(H&E stain ×200). e. High power of pervious image showing aggregates of mononuclear inflammatory cells (black arrows) surrounding necrotic hepatocytes & areas of ghost of cells and few hepatocytes with condensed/pyknotic nuclei (red arrows) (H&E stain ×400). f. Liver section from C. oil plus DM group showing normal hepatic architecture with regeneration of hepatocytes and improvement in central vein and sinusoidal blood (H&E stain ×100).
The renal tissue of the control (Fig. 3a) and C. oil (Fig. 3b) showed normal architecture with no definite histopathological alterations. The rat kidney in the group that was treated with DM showed congestion of glomerular tuft capillaries with Bowman’s capsule swelling, degeneration of renal tubules with moderate interstitial edema (Fig. 3c). Besides,the epithelial cells of renal tubules showed diffuse hydropic degenerative swelling, with dark small pyknotic nuclei (Fig. 3d) and marked diffuse interstitial edema in the medullary renal tissue with diffuse degenerative changes of distal convoluted renal tubules. (Fig. 3e). In the C. oil plus DM treated group, a mild hydropic degeneration of tubular cells was observed (Fig. 3f).
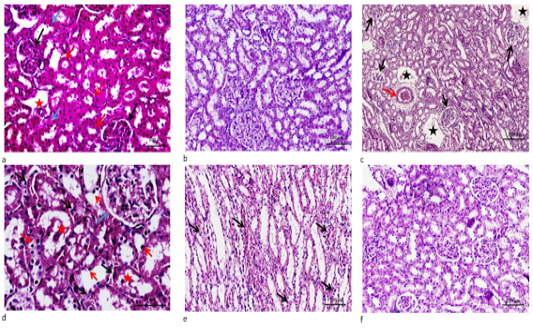
Figure 3: a. Renal section from control, showing normal glomeruli formed of capillary tuft surrounded by Bowman’s capsule ( black arrows), proximal convoluted tubules with narrow lumen lined by high cuboidal cells with homogeneous eosinophilic cytoplasm (red arrows) and distal convoluted tubules with wide lumen lined by low cuboidal cells (blue arrows) with normal- sized interstitial blood vessel (red star).(H&E stain ×200). b. Renal section from C. oil group, renal tissue demonstrated unremarkable histopathological changes. c. Renal section from DM group, cortical renal tissue demonstrated diffused moderate capillaries glomerular tuft congestion with Bowman’s capsule swelling (black arrows) note that the surrounding renal tubules showed degenerative changes with moderate interstitial edema (blue stars) and congestion (red arrow).(H&E stain ×100). d. Renal section from DM group, cortical renal tissue demonstrated diffused moderate congestion of glomerular tufts (blue arrow) & interstitial congestion (black arrows). The epithelial cells lining renal tubules showed diffuse hydropic degenerative swelling, noting some of cells had dark small pyknotic nuclei with few intraluminal sloughed cells (red stars).(H&E stain ×400). e. Renal section from DM group, medullary renal tissue demonstrated marked diffused interstitial edema and congestion with extravasation RBCS (black arrows), noting the diffuse degenertative changes of distal convoluted renal tubules (H&E stain ×200). f. Renal section from DM plus C. oil group, demonstrated mild hydropic degeneration of tubular cells and nearly normal glomeruli & interstitial tissue. (H&E stain ×200).
Discussion
According to previous studies, deltamethrin is rapidly metabolized in the liver and a great concentration of DM metabolites accumulate in the liver causing oxidative stress (Saoudi et al., 2017). In the present study, increased AST, ALT and ALP activities in DM treated rats is probably due to the overproduction of ROS that alters the oxidant-antioxidant status and disrupts the integrity of membrane lipid cell resulting in the release of hepatic enzymes from the hepatocytes into the circulation (Khalaf et al., 2017;Tewari et al., 2018). Elevation in serum AST and ALT activities after DM exposure have been reported by several studies (Abdel-Daim et al., 2013; Gündüz et al., 2015; Maalej et al., 2017).
ALT is a liver-specific enzyme and one of the most reliable indexes for hepatic damage. Elevated serum ALP activity is not restricted to hepatic damage only; it also has isoenzymes in the bones, intestines and kidneys. Therefore, the elevation in its activity may be due to injury in any of the previously mentioned organs as a result of the direct effect of the DM or as a result of oxidative damage (Uchendu et al., 2017). Moreover, the increased ALP activity in serum may be considered as an early indicator of cholestasis along with the observed hepatic cell necrosis which might be accounted for the raised level of ALP in the serum (Ikram et al., 2016).
Many previous studies revealed that pyrethroid has an impact on lipids that include triglycerides, cholesterol-free fatty acids and phospholipids (Singh and Saxena, 2002). The deltamethrin-induced increase in serum cholesterol and triglycerides levels, as observed in the present study is consistent with previous studies (Abdel-Daim et al., 2013; Ikram et al., 2016;Tewari et al., 2018).
There was no significant difference in serum total protein, albumin and globulins concentrations between the DM group and the control. Our findings are in line with Tewari et al. (2018). However, some previous reports were inconsistent with our result (Abdel-Daim et al., 2013; Uchenduet al., 2017) as they found that DM exposure resulted in decreased total protein and albumin levels. This inconsistency may be due to the different exposure time to DM, doses and conditions among the studies. In line with the present biochemical assessment results, histopathological findings demonstrated marked changes in the hepatic tissue of DM-treated rats.
The increase in serum urea concentration reflects impairment in the renal tubular reabsorption, while the elevated serum creatinine, a by-product of muscle metabolism and actively secreted by the proximal tubular cells, concentration indicates impairment of glomerular filtration rate (GFR) (Adedara et al., 2012). Therefore, the increased urea and creatinine concentrations in the DM intoxicated rats suggesting renal tissue injury (Tewari et al., 2018) which is in line with the histopathological findings.
Co-treatment with C. oil in this study was able to decline the elevated activities of AST, ALT and ALP in the C. oil plus DM group. This may be due to the antioxidant role of Cinnamon, which neutralizes the effects of the DM by scavenging of ROS. his finding was further confirmed by the improvement in the histopathological alterations and decrease the Nrf-2 gene expression in the co-exposed group.
The major constituents identified in the cinnamon oil,by GC–MS analysis of C. oil (results under publication), that have powerful antioxidant activities are cinnamaldehyde, and cinnamic acid (Bae et al., 2018). The protective potential of cinnamon oil against kidney damage induced by gentamicin was previously reported by Elkomy et al. (2020).
The transcription factor NF-E2-related factor 2 (Nrf2), is a chief mediator of the oxidative stress response through the activation of genes encoding for antioxidant enzymes (Cores et al., 2020). Nrf2 is involved in apoptosis, carcinogenesis, aging and chemical injuries (Fuse and Kobayashi., 2017).
Our findings clearly show that DM increases Nrf2 expression and that ROS production is involved as the major mediator in the DM-induced toxicity (Richardson et al., 2019). The Nrf2 gene is a novel target of DM and possibly the main coordinator of the stress response against pyrethroid toxicity (Li et al., 2011; Mohammadi et al., 2019). Former studies have shown that deltamethrin can increase ROS levels accompanied by the exhaustion of the antioxidants leading to overexpression of the Nrf-2 gene (Li et al., 2007; Kumar et al., 2015).
Furthermore, treatment with C. oil, with its potent antioxidant capacity to scavenge ROS, suppressed the DM-induced Nrf2 activation. Previous research indicated that ROS is the mediator of nuclear Nrf2 accumulation (Moretto and Colosio, 2011).
Conclusion
Co-treatment with cinnamon oil to DM-intoxicated rats significantly decreased the hepatic enzymes activities, cholesterol and triglycerides, serum urea and creatinine concentrations. Moreover, cinnamon oil efficiently lowered Nrf-2 expression and mitigated the histopathological alterations in hepatic and renal tissue induced by DM treatment.
Conflict of interest
The authors declare no conflicts of interest.
authors contribution
All authors contributed equally.
References