Advances in Animal and Veterinary Sciences
Research Article
Thymoquinone Attenuates 6-Mercaptopurine Induced Testicular Toxicity in Albino Rats: Possible Mechanisms are Involved
Naglaa Wasfey Abdelbaky1*, Ahmed Z. Abdelazem1, Khalid S. Hashem2
1Biotechnology and Life Sciences Department, Faculty of Postgraduate Studies for Advanced Sciences, Beni-Suef University, Egypt; 2Biochemistry Department, Faculty of Veterinary Medicine, Beni-Suef University, Egypt.
Abstract | The present study was conducted in order to investigate the efficacy of thymoquinone (TQ) to attenuated 6-mercaptopurine (6MP) induced testicular toxicity with insight into the mechanism(s) of protection. 6MP is an anti-cancer and immunosuppressant chemotherapeutic drug; however, it had a potent toxic effect in various body organs. Forty male adult albino rats were allocated into four equal groups: control rats, TQ(5 mg/kg.BW orally) for a month, 6MP (5 mg/kg.BW orally) for twenty days, and TQ+6MP group pretreated with TQ for ten days then co-administered with 6MP for twenty days. The oral gavage was persisted daily for 30 days followed by blood samples collection to estimate testosterone. Cauda epididymis was collected to evaluate sperm characteristics. Testes were collected to estimate histological architecture, biochemical and molecular changes of testicular tissues. 6MP treated rats showed a significant decrease in testicular weight, sperm concentration, motility and viability, serum testosterone and down-regulation in Androgen receptors (AR) mRNA, as well as, histological alteration, a significant depletion in testicular catalase and Glutathione reduced (GSH) with increasing in Malondialdehyde (MDA) levels. 6MP induced upregulation in P53 gene expression and caspase-3 activity with a down-regulation in phosphoinositide 3-kinase (PI3K). Co-treatment of TQ with 6MP improved spermatogenesis, testis histology, restoring testicular antioxidant/oxidative redox, inhibiting P53, caspase-3 apoptotic pathway and up-regulated PI3K. TQ exerts its therapeutic effect against 6MP-induced testicular damage and dysfunction through anti-oxidative and anti-apoptotic pathways.
Keywords | 6-mercaptopurine, Apoptosis, Thymoquinone, Testicular toxicity, PI3K
Received | December 23, 2019; Accepted | March 03, 2020; Published | June 02, 2020
*Correspondence | Naglaa wasfey Abdelbaky, Biotechnology and Life Sciences Department, Faculty of Postgraduate Studies for Advanced Sciences, Beni-Suef University, Egypt; Email: [email protected]
Citation | Abdelbaky NW, Abdelazem AZ, Hashem KS (2020). Thymoquinone attenuates 6-mercaptopurine induced testicular toxicity in albino rats: possible mechanisms are involved. Adv. Anim. Vet. Sci. 8(6): 653-660.
DOI | http://dx.doi.org/10.17582/journal.aavs/2020/8.6.653.660
ISSN (Online) | 2307-8316; ISSN (Print) | 2309-3331
Copyright © 2020 Abdelbaky et al. This is an open access article distributed under the Creative Commons Attribution License, which permits unrestricted use, distribution, and reproduction in any medium, provided the original work is properly cited.
Introduction
6-mercaptopurine (6MP) is an immunomodulatory and anticancer drug, clinically used in the treatment of inflammatory bowel disease, rheumatoid arthritis, autoimmune disorders and acute lymphoblastic leukemia (Morgan et al., 2015, Reggio et al., 2019). 6MP Metabolism incorporates 6- thiodeoxygoansine nucleotides into DNA, which could produce alteration in DNA-protein interaction or DNA mismatch repair. This inhibition in DNA synthesis and DNA repair system promotes the cytotoxic effects of thiopurine metabolites (Krynetski et al., 2001).
As expected for anticancer drugs the main side effects of 6MP are damaging of rapidly divided cells such as germ cells and those of bone marrow (Polifka & Friedman, 2002). Testicular atrophy, spermatogenesis impairment, and Leydig cell death are 6MP- related disorders (Morgan et al., 2015), (Schaalan et al., 2018). Recently, alternative medicine (Herbs) has been mentioned to have a promising therapeutic potential in disease treatment or in amelioration of toxicants and chemotherapy adverse effects (Ajayi et al., 2018).
Among these herbs, Black seed (Nigella sativa) is an herbaceous plant, has been used as natural antioxidants, anti-apoptotic and anti-inflammatory (Kanter et al., 2006). The most bioactive ingredient of Nigella sativa is Thymoquinone (TQ): TQ has a wide spectrum of activities and therapeutic potentials as an antioxidant, anti-inflammatory (El Gazzar et al., 2006) and anti-apoptotic (El-Ghany et al., 2009). The positive impact of TQ on spermatogenesis and fertility process have been reported previously (Mabrouk, 2018), (Salahshoor et al., 2018). Although, many reports have been conducted on TQ positive impact on the male reproductive system and its ameliorating effect against many toxicants, the efficacy of TQ against 6MP induced testicular toxicity remains unknown. Thus, in our study, we aimed to investigate the toxic effect of 6MP on a male reproductive system through evaluation of sperm characteristics and investigation of biochemical, pathological, and molecular alterations that occur in rat testis, as well as to demonstrate TQ efficacy in ameliorating testicular toxicity induced by 6MP treatment.
Material and methods
Chemicals
Thymoquinone, 6-mercaptopurine and ELISA kits were purchased from Sigma–Aldrich Company., USA. MDA and antioxidant parameters were estimated using commercial kits (Bio-diagnostic for Research Kits, Egypt). Molecular kits were obtained from QIAGEN Company, USA. Other kits and tools were purchased from high-quality sources.
Experimental Animals
This study involved 40 adult male albino rats, weighing 180-200 gram obtained from animal house, faculty of pharmacy, Beni-Suef University. Rats were transferred to the laboratory of experiments 10 days before the experiment initiation for adaptation. The laboratory conditions were maintained at a temperature of 25± 2OC and 12-hour light/day cycle with free access to water and were fed twice a day using a commercial balanced diet.
Experimental Groups
After adaptation period, rats were divided randomly into 4 equal groups (n= 10); (1) control group; rats received physiological saline orally by gastric gavage, (2)TQ group: rats received TQ (5 mg/kg BW. ) (Mabrouk, 2018) for a month, (3) 6MP group: rats received 6MP (5 mg/kg BW) for 20 days (Schaalan et al., 2018), and (4) (TQ+6MP) group: Rats pretreated with TQ only for ten days prior the co-administration with 6MP for 20 days. All doses were supplemented orally by gastric gavage.
All animal studies were conducted following the criteria of the investigations and Ethics Committee of the Community Laws (BSU-IACUC) ethical guidelines, governing the use of experimental animals of Beni-Suef University.
Blood Sampling and Tissue Preparation
24 hours post the last administered dose, rats were anesthetized using diethyl ether anesthesia, blood samples were collected from the orbital venous plexus (Parasuraman et al., 2010), and serum samples were separated for testosterone estimation. Rats were euthanized by cervical dislocation technique, testes and epididymides were carefully separated. Cauda epididymides were used for sperms characterization. Testes were washed and weighed on a microbalance, one testicle was immediately fixed in 10 % neutral formalin for histopathological examination, and the other testis was used for homogenate preparation to investigate biochemical and molecular parameters
Sperm Parameters Evaluation
Sperm characteristics were evaluated as described in (Ahmed, 2015); briefly, the cauda epididymis was isolated, chopped and immersed in 5ml of warm physiological saline then incubated at 37oC. Sperm motility was evaluated firstly; 10µl of semen solution was placed on a microscopic slide then covered, 10 films were examined using a light microscope at 40x magnification. Sperm Motility was expressed as a rate of motile and non-motile sperms and was recorded following recommendations of (Tocci & Lucchini, 2010). sperm count was obtained as described in (Yokoi et al., 2003), in brief, sperm solution was added by percentage (1:100) to solution contains 1ml formalin (35%), 25mg of Eosin, 5gm of NaHCO3 per 100 ml distal water, 10µl of the prepared solution was transferred to the counting chamber of hemocytometer using a light microscope (Sanli, China Mainland), sperms in the four corners of the central square were counted at 40x. Sperm viability and abnormality determination were followed a protocol of (Sönmez et al., 2005). 2 µl of sperm solution and 20 µl of eosin-nigrosin stain were mixed and incubated at 37oC for 15 minutes, a smear of the stained solution was examined on light microscope (40x) magnification to count dead (pink) sperms and live (unstained) sperms, around 250 sperm was examined from each sample and the ratio of viable sperms in the total count was calculated, also the abnormal morphology of sperms was counted at 40x magnification as viability counting.
Testosterone estimation: Serum testosterone levels were estimated using Enzyme Linked Immunosorbent Assay (ELISA) kit (MyBioSource Company, USA) following instructions of kits protocol (Elder & Lewis, 1985).
Oxidant/Antioxidant Assay
0.5 g of testis was homogenized in 5 ml cold saline and centrifuged at 20,000×g for 30 min at 4 °C. The supernatants were used for the assessment of MDA, GSH and catalase. Malondialdehyde ( MDA) levels were assayed according to the method of (Ohkawa et al., 1979). Catalase activity was estimated following the protocol described by (Aebi, 1984) using hydrogen peroxide substrate. Glutathione reduced (GSH) was assayed following the method of (Kum-Tatt & Tan, 1974).
Assessment of caspase-3 and pro-inflammatory marker (TNF-α): Caspase-3 activity was assayed by rat caspase-3 ELISA kit according to instructions of the manufacturer (MBS700575), MyBioSource Company, USA.
TNF-α levels: were estimated using ELISA kits according to the protocol of RayBiotech (Hopkins et al., 1988).
Western blotting assay for measuring Phosphoinositide 3-kinase (PI3K): Testicular samples were homogenized using cold RIPA lysis buffer. The lysate was incubated on ice and centrifugation at ~16,000xg for 30 minutes at 4°C. The protein concentration was determined according to instructions of Bradford Protein Assay Kit (Markham Ontario L3R 8T4 Canada) (Carlsson et al., 2011). SDS PAGE electrophoresis was used in protein separation, and then protein was transferred to nitrocellulose membrane. The membrane was incubated at 4OC overnight with anti PI3K antibodies (Thermofisher. USA), followed by incubation for I hour with the corresponding Goat anti-rabbit IgG (Deng et al., 2013). Beta-actin was used as a control. Protein band images were recorded in BioMax film and quantified using densitometry and Molecular Analyst Software (Bio-Rad, Richmond, CA, USA).
Quantitative Real time PCR (RT- qPCR): P53 mRNA and androgen receptors (AR) mRNA expression
RNA extraction: total RNA in testicular tissues was extracted and purified using RNeasy Mini Kit (Cat. No. 74104 obtained from Qiagen Company. The concentrations of purified RNA were obtained using NanoDrop spectrophotometry.
Reverse transcription reaction: 2 μg of purified RNA was used as a template for complementary DNA (cDNA) synthesis with random primers using high capacity Qiagen one-Step RT-PCR Kit (Cat .No. 205920).
Real-time qPCR reaction: was performed using (Applied Biosystems, USA) with software version 3.1 (StepOne™, USA)., gene expression technique employed following the method of (Pfaffl, 2001). The primer sequence was shown in (Table 1). PCR components include 1 µl of each primer, 5 µl of cDNA template, 5.5 µl RNAase free water and 12.5 µl cyber green mix. Running 40 cycle according to the condition of kits. Version 3.1 (StepOne™, USA) software was used in the calculation of data. The relative expression of AR and P53 was calculated using the comparative Ct method in compare to beta-actin genes. The values were reported as fold change

Table 1: Illustrated primers of P53 and Androgen receptor (AR) and the house keeping gene (Beta actin))
Histopathological Examination
The testicle was immersed immediately in 10 % neutral formalin for 48 hours. following a protocol of (Bancroft & Gamble, 2008), testis was washed, dehydrated using ethanol gradual concentration, cleared in xylene, embedded and blocked in paraffin wax. Tissues sections were microtomes on 5µ to stain by Hematoxylin and Eosin (H&E). Finally, light microscope (Olympus Pvt. Ltd., Germany) was utilized for examination of histological architecture of testis
Statistical Analysis
One-way analysis of variance (one way ANOVA) test was used in the statistical analysis of our data. All results were presented as mean ± standard error (SEM), and followed by Tukey–Kramer methods for post hoc analysis using the SPSS software program. The values of p<0.05 were considered statistically significant.
Results
Testicular Weight and Epididymal Sperm Quality
As presented in Table 2; 6MP administration induced a significant decrease in testicular weight, sperm motility %, sperm count and viability, as well as significantly increased abnormal forms of sperms in 6MP group at P<0.05, compared to the control group. On the other hand, pretreatment of TQ and its co-administration to 6MP in TQ+6MP group increased testicular weight significantly at P<0.05 in compare with 6MP group, also induced increasing in sperm count, viability and motility with a concurrent decreasing in sperm abnormalities by a significant value at P<0.05 in compare with 6MP group. There is no significant difference between control and TQ group at P<0.05.
Ar Mrna Expression and Serum Testosterone Levels
Figure 1A; Illustrated the significant decrease in AR mRNA gene expression in 6MP treated rats in comparison with the control group at P<0.05, which revealed a down-regulation ability of 6MP on ARs. Co-administered TQ in (TQ+6MP) group induced up-regulation in AR mRNA expression significantly in comparison to 6MP group.
Figure1B; Showed that 6MP treated rats had a significant decrement in testosterone level at P<0.05, compared to control group. TQ co-administration revealed a significant increase in serum testosterone levels in comparison to 6MP group at P<0.05, however, it didn’t reach the control value. There is no significant difference in testosterone and AR expression between control and TQ group at P<0.05.
Results are expressed as mean± standard error.TQ: Thymoquinone treated group, 6MP: 6-mercaptopurine treated group, TQ+6MP: co-administered of TQ and 6MP a, b superscripts in the same column, mean values with different letters are significantly difference at p˂0.05.
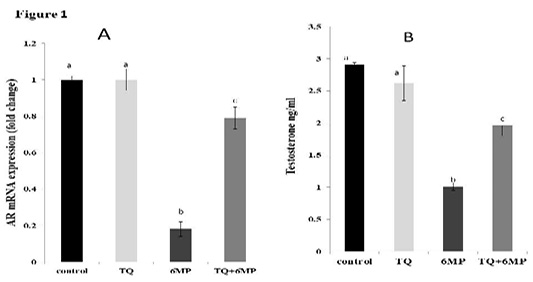
Figure 1: Effect of 6MP and TQ on Androgen receptor mRNA gene expression and serum testosterone in treated male rats.
A: Androgen receptor (AR) mRNA gene expression fold change in relative to Beta-actin
B: Serum testosterone levels in ng/ml
Values are means ± (stander error) SE
TQ: Thymoquinone treated group, 6MP: 6-mercaptopurine treated group, TQ+6MP: co-administered of TQ and 6MP
a, b, c superscripts in the same chart, values with different letters are significant difference at p˂0.05.
Testicular Antioxidants and Malondialdehyde
In Table 3; 6MP results depicted that treatment of 6MP disturbed the testicular antioxidant/oxidative redox, 6MP group showed a significant increase in MDA level with a concurrent significant depletion of testicular GSH concentration, and Catalase activities compared to the control group at P<0.05. In TQ+6MP group; TQ pretreatment restored the testicular antioxidant/ oxidative redox by increasing GSH concentration and Catalase activities, moreover, decreasing MDA level in the testis of TQ+6MP group significantly compared to 6MP group at P<0.05.

Table 3: Effect of 6MP and TQ in MDA and anti-oxidants parameters (Catalase activity and Glutathione reduced) in rat testis.
Results are expressed as mean± standard error.TQ: Thymoquinone treated group, 6MP: 6-mercaptopurine treated group, TQ+6MP: co-administered of TQ and 6MPMean values with different letters in the same column are significantly difference at p˂0.05.
P53 Mrna Expression and Caspase-3 Activity
As represented in Figure 2(A and B); 6MP increased caspase-3 activity and up-regulated P53 mRNA gene expression significantly (P<0.05) in 6MP group compared to the control. TQ administration in TQ+6MP group significantly decreased caspase-3 concentration and P53 mRNA gene expression compared to 6MP group at P<0.05. P53 mRNA of TQ+6MP group was significantly differenced with the control group at P<0.05.

Figure 2: The effect of 6MP and TQ on testicular caspase-3 and gene expression of P53 mRNA on treated rats .Values are means ± (stander error) SE
A: Represents caspase-3 values in the four treated groups
a, b superscripts in chart A, values with different letters indicated a significant difference at p˂0.05.
B: Represents P53 mRNA gene expression in treated rats, fold change in relative to Beta-actin
a, b,c superscripts in chart B, values with different letters indicated a significant difference at p˂0.05.
TQ: Thymoquinone treated group, 6MP: 6-mercaptopurine treated group, TQ+6MP: co-administered of TQ and 6MP
The Phosphoinositide 3-kinase (PI3K) Regulation
As represented in Figure 3; 6MP group had a significant decrease in PI3K protein concentration compared to the control group at P<0.05. While, co-administration of TQ results in up-regulation of PI3K in TQ+6MP significantly at P<0.05, compared to 6MP group
The Pro-Inflammatory Cytokine Tnf-Α
Figure 4 showed the significant elevation in TNF-α in 6MP group when compared to other treated groups at P<0.05. It also revealed the effect of TQ in decreasing TNF-α by a significant value in TQ+6MP compared to 6MP group, however, it did not restore TNF-α to the normal values of control group.
The most representative images of western blotting and the expression level of PI3K protein relative to Beta-actin (β-actin). Values are means ± SE
PI3K: Phosphoinositide- 3 kinase, TQ: Thymoquinone treated group, 6MP: 6-mercaptopurine treated group, TQ+6MP: co-administered of TQ and 6MP
a, b, c superscripts in the same chart, values with different letters are significant difference at p˂0.05.
The figure represents TNF-α level in all treated groups. Values are means ± SE
TNF-α: Tumor Necrosis Factor-alpha, TQ: Thymoquinone treated group, 6MP: 6-mercaptopurine treated group, TQ+6MP: co-administered of TQ and 6MP.
a, b, c superscripts in the chart, values with different letters indicated significant difference at p˂0.05.
Histopathological Results
The histopathological examination of H&E stained sections of the testicle from the examined rats revealed that the testis of control group showed the normal architecture of testicles (Figure 5a, 5b). Testicular tissue of TQ group showed also the normal seminiferous tubules having complete spermatogenesis (Figure 5c, 5d). Microscopic examination of testicles of 6MP treated rats revealed that immature germ cells were desquamated from the basal membrane, narrowing of seminiferous tubules, widening in interstitial spaces, few spermatozoa, vacuolated cytoplasm, Leydig cell atrophy, and germ cells degeneration (Figure 5e, 5f). Testis histology observed in TQ+6MP group (Figure 5g, 5h), demonstrated that seminiferous tubules were approached the normal histology of control with a reduction in germ cells losses and vacuolation. Almost cells involved in the spermatogenesis process were continued to include spermatozoa, Normal appearance of Leydig cell was observed.

Figure 5: Sections of rat testis stained with Hematoxylin and Eosin revealed the effect of 6MP and TQ in histological architecture of testicular tissues. Testis of control rats (a, b) and (TQ) Thymoquinone (c, d) shows the normal histological appearance of testis , testis of (6MP) 6-mercaptopurine treated rats (e,f) shows narrowing of seminiferous tubules, widening in interstitial spaces, few spermatozoa, germ cell degeneration. Testis of (TQ+6MP) co-administration of TQ and 6MP (g,h) shows almost normal architecture, spermatozoa and Leydig cells are histologically normal, and normal morphology of seminiferous tubules was observed.
Discussion
The efficacy of 6MP as an anticancer and immunomodulatory drug is associated with various toxicities in body systems. 6MP induced testicular damage and dysfunction have been reported previously (Schaalan et al., 2018), (Morgan et al., 2015). High mitotic activity and low antioxidant capacity of germ cells make it a target of cytotoxic agents as chemotherapeutic drugs (Rezvanfar et al., 2013). Consistent with the previous studies, in the current work, treatment of 6MP induced impairment in spermatogenesis, testicular antioxidant/oxidative redox, and triggered apoptosis. We focused on the efficacy of TQ as an anti-oxidant and anti-apoptotic agent against 6MP-induced testicular toxicity. Spermatotoxicity of 6MP was evidenced by an increasing percentage of dead sperms and abnormal morphology of sperms in 6MP treated rats with a concurrent depletion in sperm count and motility. In contrast, TQ protective effect against spermatotoxicity was evaluated by the significant increase in testicular weight, sperm concentration, and motility. Moreover, a decreased percentage of dead sperms and abnormal morphology of sperms when compared to 6MP treated group. In supporting our results (Hassan et al., 2019) reported that TQ attenuates testicular toxicity and spermatotoxicity induced by lead exposure in male rats.
6MP induced testicular atrophy was showed in the testicular weight reduction in 6MP group which could be confirmed by parenchymal atrophy, testicle lesions appeared in histopathological data which showed marked changes in testicular architecture in 6MP group when compared to other groups. 6MP induced severe degeneration and pyknosis in seminiferous tubules might attribute to oxidative stress. 6MP as a cytotoxic drug capable of producing reactive oxygen species (ROS), especially H2O2 (Kelley et al., 2010). the high concentration of polyunsaturated fatty acid on spermatozoa makes it particularly susceptible to oxidative stress and lipid peroxidation (Wathes et al., 2007). Lipid peroxidase (MDA) is an indicator of oxidative injury and has a crucial role in cytotoxicity and cellular dysfunction due to its ability in cell membrane disruption (Gutteridge, 1995). Our data clarify that 6MP induced oxidative injury in testicular tissue; testicular MDA levels highly elevated in 6MP treated rats which confirmed the ability of 6MP to disturb the hemostasis in oxidative/redox state in testicular tissue. As well as, 6MP induced a significant depletion in GSH and catalase activity in 6MP treated rats. The study of (Schaalan et al., 2018) reported the oxidative toxicity of 6MP on rat testis. Co-administration of TQ to 6MP decreased testicular MDA levels significantly in TQ+6MP group when compared to 6MP treatment alone, moreover increased the antioxidants parameters represented in GSH and catalase, this results evidenced the anti-oxidative effect of TQ against 6MP induced oxidative stress in testicular tissues (Erboga et al., 2016), (Salahshoor et al., 2018). Histopathological data of TQ+6MP group evidenced the improvement of testicular morphology was which so close to the normal architecture of the control group and confirmed the efficacy of TQ in tissue repairs and protection against oxidative injury. Supporting our data, the study of (Radad et al., 2014) reported the protective effect of TQ against brain damage, and (Gökçe et al., 2011) demonstrated the protective impact of TQ against testicular injury induced by methotrexate treatment.
6MP trigger apoptosis through P53 and caspase-3 pathway (Kanemitsu et al., 2009). The study of (Reggio et al., 2019) reported that 6MP inhibited cell proliferation by down-regulation of PI3K/AKT pathway. PI3K is a lipid kinase enzyme that play a crucial role in cell proliferation, PI3K down-regulation may induce caspase-3 activity and triggered apoptosis (Wang et al., 2017). Our data revealed that 6MP trigger testicular apoptosis via up-regulation of P53 and caspase-3 activation which could be associated with the down-regulation of PI3K observed in 6MP treated rats. In contrast, TQ co-treatment induced a significant decrease in caspase-3 concentration, P53 mRNA expression in TQ+6MP group which reflects the anti-apoptotic effect of TQ in testicular apoptosis induced by 6MP. In accordance with our finding, (Sheikhbahaei et al., 2016) reported the anti-apoptotic effect of TQ against methotrexate-induced germ cell apoptosis. TQ co-administration with 6MP induced up-regulation in PI3K by a significant value in respect to 6MP treatment alone, this result explains the ability of TQ to activate cell proliferation pathway and inhibiting apoptosis, therefore abrogate the cytotoxic effect of 6MP on testicular tissue.
Caspase-3 overexpression might induce Leydig cell death (Morgan et al., 2015). Leydig cells play a key role in testosterone production, therefore effected on Sertoli cell function (Shima et al., 2013). Serum testosterone level was remarked decreased in 6MP treated group, which was characterized by Leydig cells atrophy and germ cell degeneration. Depletion in testosterone level was associated with spermatogenesis impairment and down-regulation in AR mRNA gene expression in 6MP treated rats. Our finding was conducted with reports of (Karawya & El-Nahas, 2006) and (Kumar et al., 2006). ARs are nuclear receptors that have a crucial role in the spermatogenesis process and its knockout has an adverse effect on reproductive development and spermatogenesis (Wang et al., 2009). TQ co-treatment significantly elevation serum testosterone levels which could be attributed to the ability of TQ to diminish apoptosis and Leydig cell degeneration indicated in our results. In addition, the administration of TQ up-regulated AR gene expression, which indicated a significant elevation in AR mRNA expression values in TQ+6MP group in compared to 6MP group.
6MP treatment induced a significant elevation in TNF-α level in 6MP group, which proved the inflammatory effect of 6MP on testicular tissues. TNF-α is a pro-inflammatory cytokine that regulates multiple cellular processes in testes (Mruk & Cheng, 2004). TNF-α increment could induce spermatogenesis impairment by decreasing sperm viability and increased sperm abnormalities (Ramonda et al., 2014). 6MP co-administered with TQ showed significant depletion in TNF-α in TQ+6MP group, in compare with 6MP only, this result reflects the anti-inflammatory activity of TQ on testicular inflammation induced by 6MP.
CONCLUSION
TQ co-administration to 6MP treatment ameliorated spermatogenesis impairment and testicular toxicity induced by 6MP. TQ exerted its therapeutic potential against 6MP toxicity on testis through anti-oxidative, and anti-apoptotic.
Acknowledgement
This work is funded in part or in whole, by the scientific research developing center in Beni-suef University.
CONFLICT OF INTEREST
No conflict of interest
authors contribution
Naglaa Wasfey carried out the experiments, interpreted the results and wrote the paper manuscript. Ahmed Z. Abdelazem revised the manuscript. Khalid S. Hashem designed the protocol, supervised the experimental work, guided the results interpretation and thoroughly revised the manuscript.
References