Advances in Animal and Veterinary Sciences
Research Article
Advances in Animal and Veterinary Sciences 2 (3): 142 – 147Real–Time PCR Based Expression Study of Cyclin B Gene in Buffalo Cumulus Oocyte Complex
Jitender Yadav, Vishal Sharma, Aman Kumar, Richa Khirbat, Trilok Nanda*,
*Corresponding author: [email protected]
ARTICLE CITATION:
Yadav J, Sharma V, Kumar A, Khirbat R, Nanda T (2014). Real–Time PCR based expression study of cyclin B gene in buffalo cumulus oocyte complex. Adv. Anim. Vet. Sci. 2 (3): 142 – 147.
Received: 2013–11–20, Revised: 2013–12–16, Accepted: 2013–12–16
The electronic version of this article is the complete one and can be found online at
(
http://dx.doi.org/10.14737/journal.aavs/2014/2.3.142.147)
which permits unrestricted use, distribution, and reproduction in any medium, provided the original work is properly cited
ABSTRACT
The success rate of in vitro fertilization (IVF) in buffalo is very low, though a research of more than a decade has been carried out. One of the reasons could be the lack of standardised protocol for in vitro maturation of oocytes. The present study was conducted to determine the temporal expression of Cyclin B gene in follicles of different size. A good quality cumulus oocyte complexes (COCs) were recovered from large (>6 mm) and medium (2–6 mm) follicles of buffalo ovaries by aspiration. The recovered COCs were matured in Tissue Culture Medium–199 medium supplemented with 10% foetal bovine serum (FBS), 0.4% bovine serum albumin (BSA) and hormones pregnant mare’s serum gonadotropin (10 IU/mL) and human chorionic gonadotropins (10 IU/mL) and incubated for zero min, 30 min, 60 min, 90 min, 18 hr, 20 hr, 22 hr, 24 hr and 26 hr in a CO2 incubator with 5% CO2 under humid conditions at 38.5°C. Total RNA was isolated from COCs, reverse transcribed and real time PCR was done to study the expression pattern of Cyclin B gene in follicles of different sizes. Real time PCR assay revealed higher expression of Cyclin B in medium size follicles. However temporal expression of Cyclin B was highest at zero min in both large and medium follicles and decreases as the maturation time increases. These results suggest that the role of Cyclin B is more during early phases of maturation and less during later stages of maturation.
INTRODUCTION
In vitro maturation (IVM) and in vitro fertilization (IVF) procedures performed on oocytes obtained from slaughter–house derived ovaries have recently provided a practical means for producing large number of bovine zygotes at low cost for research and commercial purpose (Hansen, 2006). The results for IVF in buffalo are very poor as compared to cattle because majority of IVF studies in buffalo use the protocols which are established for cattle (Yousaf and Chohan, 2003). The overall success rate of embryo production following IVF in buffalo is reported to be not more than 10–15 percent which is very low when compared to that of cattle (Chauhan et al., 1998; Nandi et al., 2001; Raghu et al., 2002). Nandi et al. (2002) have reported approximately 80 percent of buffalo oocytes fail to reach the blastocyst stage and most of blastocysts are not capable of further development. The poor success rate of in vitro fertilization and lower developmental competence of in vitro produced embryos present a principal barrier for the successful implementation of other assisted reproductive techniques like animal cloning, animal transgenesis, stem cell culture and nuclear transfer etc. in buffaloes. The maturation of oocytes under in vitro condition has significant influence on the number of embryos that develop to the blastocyst stage (Thompson et al., 1995; Jones, 2000; Rizos et al., 2002). The oocyte undergoes a series of modifications during maturation and these are necessary to acquire developmental competence. Therefore more advanced research for the development of suitable in vitro maturation (IVM) system is important.
Cyclins control the transition between the phases of the cell cycle as regulatory subunits of the cyclin–dependent kinases (CDKs) (Donjerkovic and Scott, 2000). The amount of Cyclin B (which binds to Cdk1) and the activity of the Cyclin B–Cdk complex rise through the cell cycle until mitosis (Ito, 2000), where they fall abruptly due to degradation of Cyclin B (Cdk1 is constitutively present). Cyclin B protein is degraded by ubiquitin pathway (Hershko, 1999). The complex of Cdk and Cyclin B is called maturation promoting factor or mitosis promoting factor (MPF; Maller, 1990). Activated Cyclin B–Cdk complex promotes several of the events of early mitosis. The active complex phosphorylates and activates 13S condensin, which helps to condense chromosomes (Kimura et al., 1998).
Maturation–inducing hormone (MIH) induces oocytes to synthesize Cyclin B (Hirai et al., 1992; Katsu et al., 1999). The rate of Cyclin B synthesis controls the length of the first meiotic M phase (Polanski et al., 1998). Human Cyclin B protein microinjected into cycloheximide–treated bovine oocytes triggered meiotic resumption. Thus, Cyclin B has a critical role in meiotic resumption in bovine oocytes (Lévesque and Sirard, 1996). The rates of Cyclin B synthesis and degradation determine the timing of the major events taking place during meiotic maturation of the mouse oocyte (Ledan et al., 2001). GVBD in mouse oocytes is sensitive to Cyclin B abundance and the changes in distribution of Cyclin B contribute to progression through Meiosis I stage (Marangos and Caroll, 2004). Cyclin B and cdc2 (cell division control) were expressed at the mRNA level in GV oocytes but cdc2 was the only pre–MPF protein detected at that stage, thus meiosis resumption seems to be regulated by the translation of Cyclin B mRNA. Cyclin B and CDC2 mRNA were high at 10 h of IVM and decreased gradually until 26 h, where most of oocytes reached the meiosis–II stage (Khalil et al., 2010).
Thus for improving reproductive performance of buffaloes, better understanding of expression level of different genes, which have a role in meiotic resumption, is important. The present study has been conducted to determine the expression pattern of Cyclin B gene in buffalo COCs from different size follicles at different time interval during in vitro maturation.
MATERIALS AND METHODS
Collection of Ovaries
Buffalo ovaries were collected from MCD slaughter house, Ghazipur, New Delhi. All ovaries were carried to the quality control laboratory of slaughter house within 15 minutes of slaughter in normal saline solution (NSS) (at 37°C)
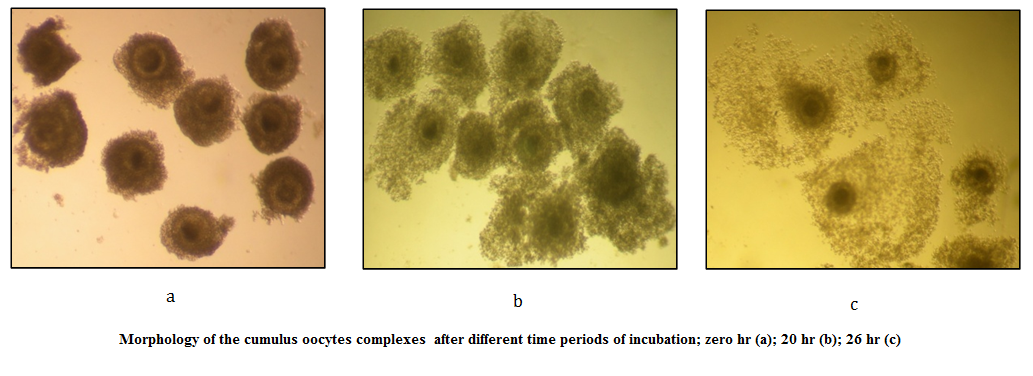
Figure 1: Morphology of the cumulus oocytes complexes after different time periods of incubation; zero hr (a); 20 hr (b); 26 hr (c)
Reverse Transcription (cDNA Synthesis); RNA Extraction and Preparation of cDNA
Total RNA was isolated from in vitro matured COCs by using TRIzol® LS reagent (Life technologies) as per the manufacturer’s instruction. Total RNA was quantified using Qubit® 2.0 fluorometer (Invitrogen). To ascertain minimum variation among different groups, a pool of 66 COCs was used for RNA isolation. Reverse transcription was carried out with total reaction volume of 20 µL. Briefly, NFW (8.00 µL), 5X RT buffer (Fermentas) (5 µL), 10mM dNTPs (Fermentas) (0.5 µL), total RNA (4 µL), M–MuLV–RT 200 IU/µL (Promega) (1 µL), DMSO (1 µL), decamer primers (Ambion) (0.5 µL). The RT–PCR cyclic conditions were as: annealing at 25oC for 10 min, reverse transcription at 42oC for 1 hour, and denaturation at 90oC for 5 min in thermal cycler (Eppendorf thermocycler). The cDNA was stored at –20oC till further use.
For the analysis of temporal expression profile of gene, real time PCR was carried out using real time PCR Applied Biosystems 7500. For the real time PCR reaction, SYBER Green dye i.e. Quantitect SYBER Green PCR kit® (Qiagen) was used and all the instructions were followed as per the supplier. The reaction for the target gene, Cyclin B gene, and the endogenous control, GAPDH gene was carried out in triplicate along with NTC (Non–template Control) as a negative control for each sample.

Figure 2: Agarose gel electropherogram of PCR product; Cyclin B (1.5%); Lane L: 100bp ladder (MBI Fermentas); Lane 1–6: PCR product of Cyclin B gene (318 bp)
The reaction mixture used to carry out the real time PCR reaction for Cyclin B and GAPDH gene a total reaction volume of 25 µL, was as: 2X Quantitect SYBER Green PCR Mix (Qiagen, 12.5 µL), primers (forward and reverse 0.3 M each), NFW (variable), 25mM MgCl2 (Fermentas, 2 µL), and template (2 µL). The cyclic conditions used for amplification of Cyclin B and GAPDH transcript cDNA were according to the instructions of the supplier. The set of primers used for the real time PCR are as shown in Table 1.
Relative Quantification by Comparative CT Method (∆∆CT Method)The average CT (Threshold cycle) value obtained for the Cyclin B (target) gene was normalized to GAPDH (endogenous control). The data obtained was subjected to comparative CT method (Livak and Schmittgen, 2001) for the analysis of the expression levels of target (Cyclin B) gene and an endogenous control. The sample at 26 hour of incubation was selected as calibrator. Cloning and Sequencing of PCR Products
In the present study the 318 bp PCR products of Cyclin B gene (Figure 2) of buffalo was used for cloning in CloneJETTM PCR Cloning Kit using pJET 1.2 blunt end cloning vector. The product was sequenced at Department of Animal Biotechnology, College of Veterinary Sciences, Lala Lajpat Rai University of Veterinary and Animal Sciences.
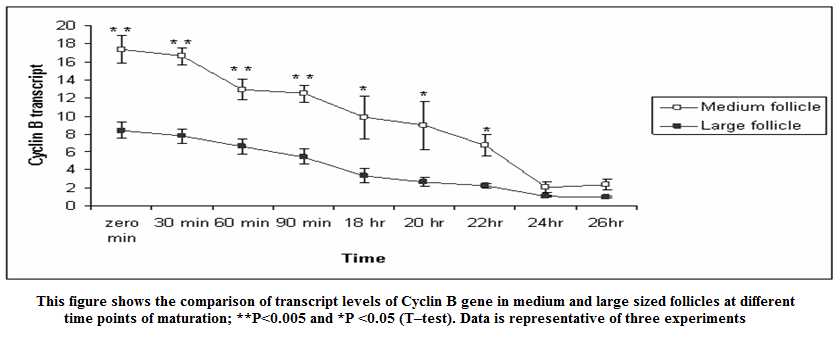
Figure 3: This figure shows the comparison of transcript levels of Cyclin B gene in medium and large sized follicles at different time points of maturation; **P < 0.005 and *P < 0.05 (T–test). Data is representative of three experiments
Phylogenetic Analysis of Nucleotide Sequences of Cyclin B
The identity analysis of the buffalo Cyclin B sequence with the other species GenBank sequence was done using BLASTn. The NCBI Genbank accession numbers of the sequences of Cyclin B of the various species considered were XR_083650.1 (Bos taurus), NM_031966.3 (Homo sapiens), XM_845305.2 (Canis lupus familiaris), XM_001491280.2 (Equus caballus), NM_001170768.1 (Sus scrofa), NM_001261149.1 (Macaca mulatta) and X60768.1 (Rattus norvegicus). The Cyclin B was found 94% identical to Bos taurus, 92% to Homo sapiens, 92% to Canis lupus familiaris, 92% to Equus caballus, 92% to Sus scrofa, 92% to Macaca mulatta and 88% to Rattus norvegicus (Table 3).
The phylogenetic tree was constructed using Phylogeny.fr server to show relatedness between the species with respect to Cyclin B fragment (Figure 4). In phylogenetic tree, Cyclin B of Bubalus bubalis were found more closely related to Bos Taurus than to Sus scrofa. From the phylogenetic tree it was observed that the Rattus norvegicus Cyclin B gene diverged much earlier in the evolution.
318 bp product length was successfully sequenced using BigDye terminator chemistry and used as Query Sequence for constructing phylogenetic tree.
DISCUSSION
Cell proliferation is an elementary cellular function and is tightly controlled by physiological signals as well as internal molecular mechanisms. In eukaryotic cells, the maturation promotion factor (MPF) is the master regulator of cell proliferation. MPF is a heterodimer of Cyclin B and cyclin dependent kinase. In this study, we report the partial sequence of buffalo Cyclin B cDNA. The identity analysis had shown that the Cyclin B gene of Bubalus bubalis is more closely related to Bos Taurus and lesser to Rattus norvegicus. Thus Rattus norvegicus Cyclin B gene has diverged much earlier in the evolution. But overall the sequence of Cyclin B has remained conserved throughout the evolution.
In present study the expression of mRNA for Cyclin B gene in in vitro matured COCs was studied by real time PCR. Our study revealed that the expression of Cyclin B gene was higher in the oocytes collected from medium sized follicles than large size follicle Majority of the oocytes completed maturation between 22 and 26 hr. These results were in agreement with those reported by Gasparrini et al. (2008), who found that the majority of buffalo oocytes accomplish nuclear maturation between 21 and 24 hr after the start of IVM. In another study, Nandi et al. (2002) found that cumulus expansion and extrusion of first polar body in buffalo oocytes commence at 6–17 hr post–maturation to reach the maximum levels at 22–24 hr. However, the results of the present study were in disagreement with the study of Neglia et al. (2001), in which the majority of buffalo oocytes reached the MII stage between 15 and 19 hr after the start of IVM and an increased incidence of degenerated oocytes was observed at later times. Large variations in the timing of the oocyte maturation process in vitro have also been reported in cattle (Ward et al., 2002; Park et al., 2005). The different oocyte maturation time–scale recorded among different buffalo studies may be accounted by different conditions of IVM and particularly by oocyte quality, which in this species, is also likely to be affected by seasonal factors.
The cytoplasmic maturation of the oocytes is a key parameter, which determines the success rate of in vitro production of embryos (Blondin and Sirard, 1995). Gaining knowledge about the variation/chronology of gene expression during oocyte maturation is crucial for optimization of IVF and other artificial reproduction technologies. Studies involving expression pattern of developmentally important genes in oocytes and embryos have not been widely investigated (Gaudette et al., 1993; Lechniak, 2002). Based on the Cyclin B mRNA transcripts in buffalo oocytes in our study, mRNA for genes that encode components of MPF were detected immediately after oocytes collection, at zero minute of IVM. Wu et al. (1997) reported that activity of MPF is low in GV–stage bovine oocytes and increases around GVBD to peak at the MI stage. In the current study, Cyclin B mRNA was high at zero minute of IVM and decreased gradually until 26 h, where most of oocytes reached the MII stage. This result correlates the resumption of meiosis with the abundance of MPF component mRNAs. The depletion of Cyclin B mRNAs could be associated with mRNA degradation or translation into MPF–component proteins.
This study is also in agreement with the results of Robert et al. (2000) who reported that bovine GV–stage oocytes, recovered from ovaries immediately after slaughter, possess a stockpile of maternal Cyclin B mRNA. Interestingly, it seems that Cyclin B protein can accumulate before oocyte maturation, when the ovary transportation time is long (Levesque and Sirard, 1996). Cyclin B mRNA probably is in a translationally inactive state (masked) in immature GV–stage bovine oocytes. Therefore, initiation of Cyclin B translation could be one of the early events leading to oocyte meiotic resumption. These studies clearly indicate that the role of Cyclin B is crucial during the early stages of folliculogenesis and its function and expression diminishes towards the later stages. Thus, the functions of Cyclin B is more conspicuous in the early phases of maturation and less during later stages of maturation of oocyte.
CONCLUSIONS
The mRNA expression of Cyclin B gene in buffalo cumulus oocyte complex was higher in medium follicles followed by large follicles. Cyclin B gene expression is found in all the IVM COCs irrespective of size of follicular source. The relative abundance of Cyclin B in COCs aspirated from graded follicles was higher just after collection i.e. at zero minute compared to those at 24 and 26 hrs of maturation. This expression pattern of Cyclin B reaffirms its role in germinal vesicle breakdown (GVBD) and early folliculogenesis and thereby proves that Cyclin B is an important factor regulating in vitro maturation of COCs.
ACKNOWLEDGEMENTS
We would like to acknowledge the help and support received from Dr. M.L. Sharma (VPH Specialist, MCD) and Dr. Senthil Kumar (Manager, Allana, Delhi) for their help for the providing the sample for our research. We would also like to acknowledge Department of Biotechnology, DBT, for the financial assistance.
REFERENCES
Blondin P, Sirard MA (1995). Oocyte and follicular morphology as determining characteristics for developmental competence in bovine oocytes. Mol. Reprod. Dev. 41: 54 – 62.
http://dx.doi.org/10.1002/mrd.1080410109
PMid:7619506
Chauhan MS, Singla SK, Palta P, Manik RS, Madan ML (1998). In vitro maturation and fertilization and subsequent development of buffalo (Bubalus bubalis) embryos: effect of oocyte quality and type of serum. Reprod. Fertil. Dev. 10: 173 – 177.
http://dx.doi.org/10.1071/R97080
PMid:9801269
Donjerkovic D, Scott DW (2000). Regulation of the G1 phase of the mammalian cell cycle. Cell Res. 10: 1 – 16.
http://dx.doi.org/10.1038/sj.cr.7290031
PMid:10765979
Gasparrini B, De Rosa A, Attanasio L, Boccia L, Di Palo R, Campanile, G, Zicarelli L (2008). Influence of the duration of in vitro maturation and gamete co–incubation on the efficiency of in vitro embryo development in Italian Mediterranean buffalo (Bubalus bubalis). Anim. Reprod. Sci. 105: 354 – 364.
http://dx.doi.org/10.1016/j.anireprosci.2007.03.022
PMid:17481834
Gaudette MF, Cao QP, Crain WR (1993). Quantitative analysis of specific messenger RNAs in small numbers of preimplantation embryos. Methods Enzymol. 225: 328 – 344.
http://dx.doi.org/10.1016/0076-6879(93)25022-T
Hansen PJ (2006). Realizing the promise of IVF in cattle – an overview. Theriogenol. 65: 119 – 125.
http://dx.doi.org/10.1016/j.theriogenology.2005.09.018
http://dx.doi.org/10.1016/j.theriogenology.2005.09.019
PMid:16253320
Hershko A (1999). Mechanisms and regulation of the degradation of cyclin B. Philos. Trans. R. Soc. Lond. B. Biol. Sci. 354: 1571 – 1576.
http://dx.doi.org/10.1098/rstb.1999.0500
PMid:10582242 PMCid:PMC1692665
Hirai T, Yamashita M, Yoshikuni M, Lou YH, Nagahama Y (1992). Cyclin B in fish oocytes: its cDNA and amino acid sequences, appearance during maturation, and induction of p34cdc2 activation. Mol. Reprod. Dev. 33: 131 – 140.
http://dx.doi.org/10.1002/mrd.1080330204
PMid:1418982
Ito M (2000). Factors controlling cyclin B expression. Plant Mol. Biol. 43: 677 – 690.
http://dx.doi.org/10.1023/A:1006336005587
PMid:11089869
Jones GM (2000). Growth and viability of human blastocysts in vitro. Reprod. Med. Rev. 8: 203 – 249.
http://dx.doi.org/10.1017/S0962279900000351
Katsu Y, Yamashita M, Nagahama Y (1999). Translational regulation of cyclin B mRNA by 17–alpha, 20–beta–dihydroxy–4–pregnen–3–one (maturation–inducing hormone) during oocyte maturation in a teleost fish, the goldfish (Carassius auratus). Mol. Cell Endocrinol. 158: 79 – 85.
http://dx.doi.org/10.1016/S0303-7207(99)00177-X
Khali WKB, Alam SS, Barakat IAH, Hassan AM, Mahrous KF (2010). Effect of in vitro culture on the expression of genes enhanced meiotic progression in Egyptian buffalo oocytes. J. App. Biosci. 32: 1977 – 1988.
Kimura K, Hirano M, Kobayashi R, Hirano T (1998). Phosphorylation and activation of 13S condensin by CDC2 In Vitro. Sci. 282: 487 – 490.
http://dx.doi.org/10.1126/science.282.5388.487
Lechniak D (2002). Quantitative aspect of gene expression analysis in mammalian oocytes and embryos. Reprod. Biol. 2: 229 – 241.
PMid:14666147
Ledan E, Polanski Z, Terret ME, Maro B (2001). Meiotic maturation of the mouse oocyte requires equilibrium between cyclin B synthesis and degradation. Dev. Biol. 23: 400 – 413.
http://dx.doi.org/10.1006/dbio.2001.0188
PMid:11401401
Levesque JT, Sirard MA (1996). Resumption of meiosis is initiated by the accumulation of cyclin B in bovine oocytes. Biol. Reprod. 55: 1427 – 1436.
http://dx.doi.org/10.1095/biolreprod55.6.1427
PMid:8949903
Livak KJ, Schmittgen TD (2001). Analysis of relative gene expression data using real time quantitative PCR and the 2–∆∆Ct method. Meth. 25: 402 – 408.
http://dx.doi.org/10.1006/meth.2001.1262
PMid:11846609
Maller JL (1990). MPF and cell cycle control. Adv. Second Messenger Phosphoprotein Res. 24: 323 – 328.
PMid:2169812
Marangos P, Carroll J (2004). The dynamics of cyclin B1 distribution during meiosis I in mouse oocytes. Reprod. 128: 153 – 162.
http://dx.doi.org/10.1530/rep.1.00192
PMid:15280554
Nandi S, Ravindranatha BM, Gupta PSP, Sharma PV (2001). Effect of somatic cells monolayer on maturation of buffalo oocytes in vitro. Ind. J. Anim. Sci. 71: 936 – 937.
Nandi S, Raghu HM, Ravindranatha BM, Chauhan MS (2002). Production of buffalo (Bubalus bubalis) embryos in vitro: premises and promises. Reprod. Domest. Anim. 37: 65 – 74.
http://dx.doi.org/10.1046/j.1439-0531.2002.00340.x
PMid:11975742
Neglia G, Marino M, Di Palo R, Wilding M, Caracciolo di Brienza V, Dale B, Gasparrini B, Zicarelli L (2001). A comparison of in vitro maturation in buffalo (Bubalus Bubalis) and bovine oocytes using confocal microscopy. Theriogenol. 55: 488.
Park YS, Kim SS, Kim JM, Park HD, Byun MD (2005). The effects of duration of in vitro maturation of bovine oocytes on subsequent development, quality and transfer of embryos. Theriogenol. 64: 123 – 134.
http://dx.doi.org/10.1016/j.theriogenology.2004.11.012
PMid:15935848
Polanski Z, Ledan E, Brunet S, Louvet S, Verlhac MH, Kubiak JZ, Maro B (1998). Cyclin synthesis controls the progression of meiotic maturation in mouse oocytes. Develop. 125: 4989 – 4997.
Raghu HM, Reddy SM, Nandi S (2002). Effect of insulin, selenium and epidermal growth factor on development of buffalo oocytes to the blastocyst stage in in vitro with serum free and semi defined media. Vet. Rec. 151: 260 – 265.
http://dx.doi.org/10.1136/vr.151.9.260
PMid:12233827
Rizos D, Ward F, Duffy P, Boland MP, Lonergan P (2002). Consequence of bovine oocyte maturation, fertilization or early embryo development in vitro versus in vivo: implications for blastocyst yield and blastocyst quality. Mol. Reprod. Dev. 61: 234 – 248.
http://dx.doi.org/10.1002/mrd.1153
PMid:11803560
Robert C, Barnes FL, Hue I, Sirard MA (2000). Subtractive hybridization used to identify mRNA associated with the maturation of bovine oocytes. Mol. Reprod. Dev. 57: 167 – 175.
http://dx.doi.org/10.1002/1098-2795(200010)57:2<167::AID-MRD8>3.0.CO;2-P
Thompson JG, Gardner DK, Pugh PA, Mc Millan WH, Tervit HR (1995). Lamb birth weight is affected by culture system utilized during in vitro pre–elongation development of ovine embryos. Biol. Reprod. 53: 1385 – 1391.
http://dx.doi.org/10.1095/biolreprod53.6.1385
PMid:8562695
Ward F, Enright B, Rizos D, Boland M, Lonergan P (2002). Optimization of in vitro bovine embryo production: effect of duration of maturation, length of gamete co–incubation, sperm concentration and sire. Theriogenol. 57: 2105 – 2117.
http://dx.doi.org/10.1016/S0093-691X(02)00696-9
Wu B, Ignotz G, Currie WB, Yang X (1997). Dynamics of maturation–promoting factor and its constituent proteins during in vitro maturation of bovine oocytes. Biol. Reprod. 56: 253 – 259.
http://dx.doi.org/10.1095/biolreprod56.1.253
PMid:9002657
Yousaf MR, Chohan KR (2003). Nuclear morphology, diameter and meiotic competence of buffalo oocytes relative to follicle size. Reprod. Fertil. Dev. 15: 223 – 229.
http://dx.doi.org/10.1071/RD03006
PMid:12921697