Advances in Animal and Veterinary Sciences
Research Article
Influence of Biochar and Liquid Smoke Additives from Cacao-Pod Husks on In vitro Ruminal Fermentation Characteristics
Novia Qomariyah1,2,5,*, Yuli Retnani3*, Anuraga Jayanegara3,5, Elizabeth Wina4, Idat G. Permana3
1Graduate School of Nutrition and Feed Science, Faculty of Animal Science, IPB University, Bogor 16680, Indonesia; 2South Sulawesi Assessment Institute for Agricultural Technology (South Sulawesi AIAT), Makassar 90243, Indonesia; 3Department of Nutrition and Feed Technology, Faculty of Animal Science, IPB University, Bogor 16680, Indonesia; 4Indonesian Research Institute for Animal Production, Ciawi Bogor 16720, Indonesia; 5Animal Feed and Nutrition Modelling (AFENUE) Research Group, Faculty of Animal Science, IPB University, Bogor 16680, Indonesia.
Abstract | The objective of this study was to investigate the influence of biochar (BC) and liquid smoke (LS) additives from cacao pod husks on in vitro ruminal fermentation products, ruminal microbiota and feed degradation. The in vitro effects of different levels of BC and LS on rumen fermentation characteristics were assessed in a randomized block design experiment with five treatments and four replicates (BC and LS were separately analyzed). The treatments consisted of a basal ration (forage: pollard; 60:40 w/w) with various BC additions: BC0 (basal ration + 0 mg/ml biochar), BC1 (basal ration + 0.1 mg/ml biochar), BC2 (basal ration + 0.2 mg/ml biochar), BC3 (basal ration + 0.3 mg/ml biochar), and BC4 (basal ration + 0.4 mg/ml biochar) and LS additions: LS0 (basal ration + 0 µl/ml liquid smoke), LS1 (basal ration + 0.25 µl/ml liquid smoke), LS2 (basal ration + 0.5 µl/ml liquid smoke), LS3 (basal ration + 0.75 µl/ml liquid smoke), and LS4 (basal ration + 1 µl/ml liquid smoke). These treatments were subjected to in vitro incubation with buffered rumen fluid for 48 hours. Results showed that the addition of BC and LS did not affect pH, ammonia, or total gas production. Compared to the BC0 treatment, BC addition significantly increased acetate production (P < 0.01), and significantly decreased butyrate, isobutyrate, valerate, and isovalerate (P < 0.05). Compared to the LS0 treatment, LS addition significantly increased total volatile fatty acids (VFA), acetate, and acetate to propionate ratio (P < 0.05). Biochar addition above 0.1 mg/ml but not LS addition significantly decreased (P < 0.01) in vitro ruminal dry and organic matter degradabilities. Biochar addition above 0.1 mg/ml, but not LS addition significantly decreased total bacteria population (P < 0.001). Addition of BC above 0.2 mg/ml decreased protozoa population (P < 0.001) while LS decreased protozoa population, dependent on dose (P < 0.001). In conclusion, biochar and liquid smoke from cacao-pod husks had different effects on ruminal fermentation characteristics. However, both of them partially defaunated ruminal microbiota and shift VFA composition.
Keywords | Biochar, Liquid Smoke, Fermentation, Rumen, In Vitro
Received | December 05, 2020; Accepted | December 16, 2020; Published | February 20, 2021
*Correspondence | Novia Qomariyah, Yuli Retnani, Graduate School of Nutrition and Feed Science, Faculty of Animal Science, IPB University, Bogor 16680, Indonesia; Department of Nutrition and Feed Technology, Faculty of Animal Science, IPB University, Bogor 16680, Indonesia; Email: [email protected]; [email protected]
Citation | Qomariyah N, Retnani Y, Jayanegara A, Wina E, Permana IG (2021). Influence of biochar and liquid smoke additives from cacao-pod husks on in vitro ruminal fermentation characteristics. Adv. Anim. Vet. Sci. 9(4): 533-543.
DOI | http://dx.doi.org/10.17582/journal.aavs/2021/9.4.533.543
ISSN (Online) | 2307-8316; ISSN (Print) | 2309-3331
Copyright © 2021 Qomariyah et al. This is an open access article distributed under the Creative Commons Attribution License, which permits unrestricted use, distribution, and reproduction in any medium, provided the original work is properly cited.
INTRODUCTION
The use of antibiotics in animal feed is often aimed at improving growth performance and preventing the adverse effects of pathogens and non-pathogens. However, this use has been prohibited in many countries over the last two decades. The prohibition of antibiotic use is attributed to increasing antibiotic resistance in humans and animals, especially in Salmonella spp. and Escherichia coli (Qomariyah et al., 2019). Antibiotics can increase the weight, growth, and performance of livestock, but if used continuously, antibiotics cause microbial resistance to livestock and have residues for livestock and humans (Marshall and Levy, 2011). The use of antibiotics as growth promoters has been banned by the European Union since 2006 (EC Regulation No. 1831/20031) because of their negative effects on animal health and food safety (European Food Safety Authority, 2009). This prohibition has led farmers to switch to natural non-antibiotic alternative ingredients such as prebiotics, probiotics, organic acids, phytogenics, and zeolites which improve the health and growth performance of livestock (Papatsiros et al., 2012). The use of these materials has disadvantages, such as low potency, expensive production costs, and short-term effects.
Given these issues, to minimize or eliminate pathogenic infections and colonization in livestock it is necessary to develop new strategies and commercial products that are effective, cheap, and environmentally friendly. One of the plants sources of alternative bioactive compounds is cacao (Theobroma cacao). At present, Indonesia is the third largest producer of cacao, at 777,750 MT/year (FAOSTAT, 2014). Cacao beans are an industrially important crop because cacao is the key ingredient of chocolate, one of the most common foods in the world, and its processed goods. However, waste in the form of cacao-pod husks is also produced (Mansur et al., 2014). Cacao fruit has four component parts: (a) 73.7% pod husk; (b) 10.1% pulp; (c) 2.0% placenta; and (d) 14.2% seeds (Watson et al., 2012; Young, 2007). The cacao-pod husks contain crude fiber as their main component (55.7%) (Laconi and Jayanegara, 2015) and this fiber contains 35.4% cellulose, 37% hemicellulose, and 14.7% lignin (Daud et al., 2013). The use of this biomass to increase added value can be carried out by physical, chemical, and biological processing (Lehmann and Joseph, 2015).
Pyrolysis is one of the physical processes used to produce low-cost and environmentally friendly compounds. Pyrolysis is a thermal decomposition process in which, in the absence of oxygen, biomass is heated at moderate to high temperatures, resulting in the conversion of biomass into three main products: carbon-rich solid residues (biochar), the liquid condensation portion of the released volatile matter (liquid smoke) and the remaining non-condensable gases, such as CO, CO2, CH4 and H2 (Kambo and Dutta 2015; Liu and Wang 2018). Biochar compounds are porous and have a large surface area (Hernandez-Mena et al., 2014; Tomczyk et al., 2020) that allows them to absorb gases and carbon, bind to toxins, and provide a biofilm habitat in which microbiota can reproduce (Hansen et al., 2012; Lehmann and Joseph, 2015). Biochar also exhibits electron-mediating properties in biological redox reactions (Yu et al., 2015) and provides benefits in the form of feed efficiency in ruminants and the reduction of greenhouse gas emissions (Leng et al., 2012a). The phenolic compounds contained in liquid smoke are known to be antimicrobial agents (Beker et al., 2016) and to also have antioxidant properties (Crozier et al., 2011). Liquid smoke from cashew-nut shells can reduce the number of E. coli bacteria in an in vitro trial (Sinurat et al., 2018). The supplementation of 1% bamboo vinegar powder in feed ration provides an immune effect similar to antibiotics (Qin et al., 2018). The use of biochar and liquid smoke from cacao-pod husks is expected to support antibiotic-free programs in which farmers are directed to utilize the functional ingredients in their surroundings as natural and environmentally friendly growth-stimulating agents.
This study aimed to investigate the influence of biochar (BC) and liquid smoke (LS) additives from cacao pod husks on in vitro ruminal fermentation products, ruminal microbiota and feed degradation.
MATERIALS AND METHODS
Ethical Approval
The experiment was approved by the Animal Welfare Commission of the Indonesian Agency for Agricultural Research and Development (Balitbangtan/BPTP Sulsel/Rm/02/2020) and was conducted at the Laboratory of Dairy Nutrition, IPB University, Indonesia. This study used the rumen liquor from one ruminally non-lactating Holstein dairy cow located at the field experimental station, Faculty of Animal Science, IPB University, Indonesia. Animal were cared for according to the IPB University standard for animal welfare.
Sample Preparation
Cacao-pod husks were obtained from a cacao plantation in Bogor Regency, West Java Province. The fresh cacao pod husks were chopped and dried and then processed at the Central Laboratory for Forest Products Research and Development, Bogor, and BC and LS were obtained as pyrolysis products. The chemical composition and physical properties of the BC and LS used can be seen in Table 1. Basal rations (based on dry matter) consisted of 60% grass and 40% pollard. The basal ration was composed to resem
Table 1: Chemical composition and physical properties of biochar and liquid smoke used*
Component | Biochar | Component | Liquid Smoke |
Moisture (%) | 7.69 | Moisture (%) | 61.9 |
Ash (%) | 17.2 | pH | 4.80 |
Ca (%) | 1.70 | Total phenol (%) | 3.12 |
P (%) | 0.052 |
Bulk density (g/cm3) |
1.015 |
Mg (%) | 0.304 | Total organic acid (%) | 34.3 |
K (%) | 2.40 | ||
Fe (ppm) | 2374 | ||
Zn (ppm) | 27 | ||
Cu (ppm) | 30 | ||
pH | 10.7 | ||
Volatile matter (%) | 23.8 | ||
Fixed carbon (%) |
59.0 |
Table 2: Chemical composition and nutrient content (%DM)
Feed Ingredient |
Ash (%) |
CP (%) |
EE (%) |
CF (%) | NFE (%) |
NDF (%) |
ADF (%) |
TDN (%) |
Ca (%) |
P (%) |
Pollard | 5.40 | 15.88 | 2.05 | 3.36 | 55.64 | 55.23 | 15.64 | 66.99 | 0.005 | 0.082 |
Forage | 6.77 | 11.20 | 4.09 | 20.42 | 44.59 | 74.07 | 43.97 | 54.87 | 0.684 |
0.084 |
CP : crude protein, EE : ether extract; CF : crude fiber; NFE : Nitrogen free extract; NDF : neutral detergent fiber; ADF : acid detergent fiber; TDN : total digestible nutrient
ble rations used in Cordero Farm (a goat farm), Bogor. The nutritional content of the basal ration can be seen in Table 2. Chemical composition was analyzed in duplicate according to the AOAC method (Association of Official Analytical Chemists, 2005).
In Vitro Incubation Procedure
The sample was evaluated for in vitro ruminal fermentation and degradability using the procedure used by Theodorou et al. (1994). The in vitro incubation was performed in four consecutive runs (replicates) at different weeks, in which each sample per run was represented by six fermentation tubes. Approximately 750 mg of sample was placed into a serum bottle (100 ml capacity) and 25 ml ruminal fluid and 50 ml bicarbonate buffer were added. All serum bottles were exposed to CO2 for 30 s and then closed with a butyl rubber plug and aluminum crimp seal to begin incubation in a water bath at 39°C. Four tubes were removed after 24 h incubation and the remaining tubes were continued for another 48 h incubation. Gas was released and recorded regularly at 2, 4, 6, 8, 10, 12, and 24 hours incubation using a 100 mL syringe. Shaking was performed manually each time gas was taken. After the first stage of incubation (24 h), the tubes were centrifuged at 3500 rpm for 15 min. The supernatant (fermentation fluid) was separated and sampled for pH, ammonia concentration, and partial VFAs. Ammonia concentration was determined by the Conway micro-diffusion method (General Laboratory Procedure, 1966). Partial VFA concentration was analyzed by gas chromatography (Bruker Scion 436 GC) with a capillary column BR-Wax fame containing WCOT of fused silica with a column length of 30 m x 0.32 mm. Ionization detection was used to evaluate the VFA products of fermentation. Nitrogen 25 ml/min was used as the carrier gas and hydrogen 30 mL/min as the combustion gas. The temperature of the injector was 250ºC, and in 11 minutes, the column temperature gradient was 70–150ºC. The flame ionization detector (FID) temperature of the detector used was 275°C. Gas-production kinetics was calculated through the exponential equation (Orskov and McDonald, 1979) as follows:
p = a + b (1 – e– ct)
where p is the cumulative gas production at t hours, while a, b, and c are constants of the exponential equation. The constants a, b, and c can be interpreted as the production of gas from the soluble fraction (a), the production of gas from the insoluble but fermentable fraction (b), and the rate of reaction of gas formation (c). Thus a + b can be interpreted as the maximum gas production that can be formed during the fermentation process at time t approaches infinity (Orskov and McDonald, 1979). Biochar and liquid smoke were separately analyzed. The calculations were carried out using a nonlinear regression equation in SPSS Version 20 software.
Gas samples were then injected into a gas chromatograph to measure methane concentration, using a Shimadzu 14A GC with an Flame Ionization Detector (FID). The total bacterial population was analyzed using Ogimoto and Imai’s (1981) methods. The protozoa populations in the rumen fluid were calculated using a hemocytometer with methyl-green formalin saline solution (Ogimoto and Imai, 1981). In the second stage of in vitro fermentation, the supernatants in the remaining tubes were discarded after centrifugation (3500 rpm for 15 min). The residue was analyzed for dry matter (DM) and organic matter (OM) to identify in vitro ruminal dry matter degradability (IVRDMD) and in vitro ruminal organic matter degradability (IVROMD) values (Tilley and Terry, 1963).
Experimental Design and Data Analysis
This study used a randomized block design with five treatments and four replications (BC and LS were analyzed separately). The treatments consisted of a basal ration (forage:pollard, 60:40 w/w) with additions as follows: BC0 (basal ration + 0 mg/ml biochar), BC 1 (basal ration + 0.1 mg/ml biochar), BC2 (basal ration + 0.2 mg/ml biochar), BC3 (basal ration + 0.3 mg/ml biochar), BC4 (basal ration + 0.4 mg/ml biochar), and LS additions: LS0 (basal ration + 0 μl/ml liquid smoke), LS1 (basal ration + 0.25 µl/ml liquid smoke), LS2 (basal ration + 0.5 µl/ml liquid smoke), LS3 (basal ration + 0.75 µl/ml liquid smoke), and LS4 (basal ration + 1 µl/ml liquid smoke). Different in vitro processes were divided into groups due to differences in the population variations and ruminal microbial activity in each sampling time. Detected outlier data were removed from the data set when their Z score was lower than -2 or higher than 2. Data were analyzed using ANOVA at a 5% significance level. The comparison between the different treatments was analyzed using Duncan’s multiple range test at a 5% significance level. Data analysis was conducted using IBM SPSS Statistics version 20.
RESULTS
The addition of biochar (BC) and liquid smoke (LS) did not affect pH, ammonia, total gas or methane production (Table 3). The in vitro gas-production kinetics with BC and LS additions during the 24 h incubation period can be seen in Figures 1 and 2, respectively. In both, it can be seen that there was a quite large increase in gas production in the first 8 h of incubation, then it decreased along with the fermentation period. Compared to the BC0 treatment, BC addition tended to increase total VFA production (P < 0.1) and significantly increased acetate production (P < 0.01). However, increasing the dose of BC did not cause any significant difference in acetate production. Addition of BC significantly reduced butyrate, isobutyrate, valerate and isovalerate production (P < 0.05), however, there was no significant difference in their values with increasing levels of BC.
Compared to the LS0 treatment, LS addition significantly increased total VFAs, acetate, and acetate to propionate
Table 3: In vitro total gas production, gas production kinetics and methane concentration of basal ration added with biochar (BC) and liquid smoke (LS).
Treatment | Level | pH |
Gas 24 h (ml) |
Gas 48 h (ml) |
a + b (ml) |
c (ml/h) |
CH4 (mmol/ml gas) |
N-NH3 (mM) |
Biochar (mg/ml) : | ||||||||
BC0 | 0 | 7.11 | 74.9 | 107.1 | 124.6 | 0.043 | 4.53 | 5.66 |
BC1 | 0.1 | 6.97 | 78.6 | 111.7 | 127.8 | 0.044 |
4.55 |
4.10 |
BC2 | 0.2 | 6.98 | 77.2 | 109.5 | 125.3 | 0.045 | 4.47 | 4.81 |
BC3 | 0.3 | 6.97 | 76.4 | 108.5 | 122.6 | 0.045 | 4.78 | 5.03 |
BC4 | 0.4 | 6.93 | 75.9 | 108.5 | 124.3 | 0.044 | 4.42 | 4.66 |
SEM | 0.03 | 2.31 | 1.88 | 1.74 | 0.002 | 0.07 | 0.35 | |
P-Value | 0.248 | 0.286 | 0.287 | 0.277 | 0.625 | 0.571 | 0.399 | |
Liquid smoke (µl/ml) : | ||||||||
LS0 | 0 | 7.11 | 74.9 | 107.1 | 124.6 | 0.043 | 4.53 | 5.66 |
LS1 | 0.25 | 7.05 | 80.9 | 111.2 | 122.4 | 0.051 | 4.87 | 4.45 |
LS2 | 0.5 | 7.05 | 83.4 | 113.9 | 124.1 | 0.053 | 4.86 | 4.47 |
LS3 | 0.75 | 6.98 | 78.5 | 111.9 | 129.1 | 0.044 | 4.51 | 4.93 |
LS4 | 1 | 6.91 | 78.0 | 110.7 | 122.5 | 0.046 | 4.79 | 5.36 |
SEM | 0.03 | 2.43 | 2.17 | 2.33 | 0.002 | 0.07 | 0.31 | |
P-Value | 0.160 | 0.484 | 0.608 | 0.614 | 0.154 | 0.173 | 0.622 |
a+b : maximum gas production; c : gas production rate
Table 4: In vitro ruminal fermentation parameters of basal ration added with biochar (BC) and liquid smoke (LS)
Treatment | Level | Total VFA (mM) | VFAs (% molar proportion) | C2:C3 | |||||
C2 | C3 | C4 | isoC4 | C5 |
isoC5 |
||||
Biochar (mg/ml) : | |||||||||
BC0 | 0 | 52.74 |
47.95b |
21.80 |
12.26a |
8.05a |
4.09a |
5.85a |
2.23 |
BC1 | 0.1 | 72.32 |
54.89a |
21.24 |
11.41ab |
5.77b |
2.24bc |
4.43b |
2.72 |
BC2 | 0.2 | 84.48 |
57.67a |
21.64 |
10.27b |
5.21b |
1.69c |
3.51b |
2.74 |
BC3 |
0.3 | 63.21 |
56.08a |
20.40 |
10.48b |
6.03b |
2.71b |
4.29b |
2.77 |
BC4 | 0.4 | 67.29 |
58.03a |
20.26 |
10.01b |
5.75b |
2.03bc |
3.91b |
2.93 |
SEM | 5.05 | 1.33 | 0.68 | 0.38 | 0.29 | 0.25 | 0.24 | 0.13 | |
P-Value | 0.074 | 0.01 | 0.94 | 0.037 | 0.009 | 0.001 | 0.005 | 0.384 | |
Liquid smoke (ul/ml) : | |||||||||
LS0 | 0 |
52.75b |
47.95b |
21.80 | 12.26 |
8.05a |
4.08 |
5.85a |
2.23b |
LS1 | 0.25 |
68.47ab |
57.40a |
19.90 | 10.64 |
5.71b |
2.28 |
4.06b |
2.89a |
LS2 | 0.5 |
68.24a |
55.35a |
19.20 | 11.44 |
6.51b |
2.74 |
4.76ab |
2.89a |
LS3 | 0.75 |
65.33a |
56.55a |
18.59 | 10.79 |
6.43b |
2.95 |
4.68ab |
3.07a |
LS4 | 1 |
61.80a |
58.66a |
18.39 | 10.90 |
5.77b |
2.07 |
4.21b |
3.21a |
SEM | 2.82 | 1.25 | 0.45 | 0.31 | 0.29 | 0.29 | 0.25 | 0.11 | |
P-Value | 0.034 | 0.002 | 0.156 | 0.083 | 0.014 | 0.06 | 0.033 |
0.03 |
C2: acetate; C3: propionate: C4: butyrate; C5: valerate.
a-b : means in the same column with varying superscript differ significantly (P<0.05)
Table 5: In vitro ruminal degradability, total bacteria and protozoa population of basal ration added with biochar (BC) and liquid smoke (LS).
Treatment | Level | IVRDMD (%) | IVROMD (%) |
Total Bacteria Population (log10/ml) |
Total Protozoa Population (log10/ml) |
Biochar (mg/ml) : | |||||
BC0 | 0 |
43.93a |
46.56a |
9.53a |
6.77a |
BC1 | 0.1 |
44.33a |
45.47ab |
9.49ab |
6.71a |
BC2 | 0.2 |
41.91b |
43.69bc |
9.47b |
6.66a |
BC3 | 0.3 |
41.95b |
42.72cd |
9.41c |
6.49b |
BC4 | 0.4 |
40.86b |
41.16d |
9.38c |
6.25c |
SEM | 0.56 | 0.74 | 0.01 | 0.05 | |
P-Value | 0.003 | <0.001 | <0.001 | <0.001 | |
Liquid smoke (ul/ml) : | |||||
LS0 | 0 | 43.93 | 46.56 | 9.46 |
6.77a |
LS1 | 0.25 | 45.95 | 48.26 | 9.36 |
6.69b |
LS2 | 0.5 | 45.09 | 47.38 | 9.36 |
6.61c |
LS3 | 0.75 | 46.56 | 48.89 | 9.38 |
6.51d |
LS4 | 1 | 45.27 | 47.45 | 9.39 |
6.38e |
SEM | 0.45 | 0.46 | 0.02 | 0.04 | |
P-Value | 0.226 | 0.230 | 0.527 |
<0.001 |
a-e : means in the same column with varying superscript differ significantly (P<0.05);
IVRDMD: in vitro ruminal dry matter degradability; IVROMD: in vitro ruminal organic matter degradability
ratio (P < 0.05). However, increasing the level of LS addition did not cause any significant difference in total VFA, acetate, and acetate to propionate ratio. Addition of LS significantly decreased isobutyrate and isovalerate production (P < 0.05); however, no significant difference in their values with increasing level of LS was seen. The production
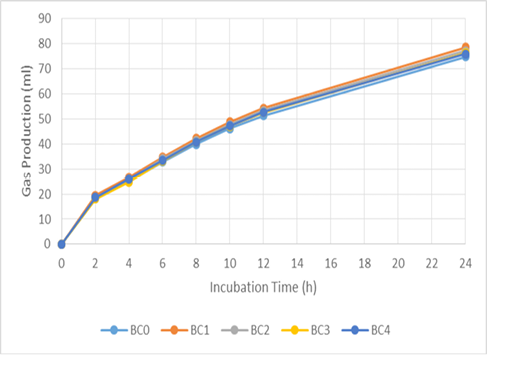
Figure 1: Cumulative gas production of basal ration added with biochar (BC) during 24 hours incubation. BC0 (basal ration+0 mg/ml biochar), BC 1 (basal ration+0.1 mg/ml biochar), BC2 (basal ration+0.2 mg/ml biochar), BC3 (basal ration+0.3 mg/ml biochar), BC4 (basal ration+0.4 mg/ml biochar)

Figure 2: Cumulative gas production of basal ration added with liquid smoke (LS) during 24 hours incubation. LS0 (basal ration+ 0 μl/ml liquid smoke) is added before LS1 (basal ration+ 0.25 μl/ml liquid smoke), LS2 (basal ration+0.5 µl/ml liquid smoke), LS3 (basal ration+0.75 µl/ml liquid smoke), LS4 (basal ration+1 µl/ml liquid smoke).
of butyrate and valerate tended to decrease (P < 0.1) with addition of LS.
In vitro ruminal dry matter and organic matter degradabilities (IVRDMD and IVROMD) decreased significantly with BC addition above 0.1 mg/ml, while non-significant difference in IVRDMD and IVROMD were seen with LS addition.
Biochar addition above 0.1 mg/ml significantly decreased total bacteria population (P < 0.001) but this was not the case for LS addition. Addition of BC above 0.2 mg/ml decreased protozoa population (P < 0.001) while LS addition produced dose-dependent decreased protozoa population (P < 0.001).
DISCUSSION
Gas production in the rumen is derived from the fermentation of feed by various microbes. Volatile fatty acid (VFA), carbon dioxide (CO2), and hydrogen (H2) molecules are produced during the fermentation of carbohydrates by bacteria, protozoa, and fungi in the rumen. Volatile fatty acids function as the main energy source for ruminants (Vyas et al., 2016). Furthermore, overall production of gas provides a representation of nutrient profiles (Wahyono et al., 2019). The in vitro gas production rate for each treatment decreased with increased incubation time, due to the reduction in the amount of easily fermented substrate. Jayanegara and Sofyan (2008) attributed the decrease in the rate of gas production as being related to VFA production indicating a reduced energy supply for livestock. The positive correlation between incubation time and in vitro gas production is associated with degradation of the incubated feed samples over a longer incubation time (Maccarana et al., 2016). Previous research has shown similar results, in which there were no differences in gas production when BC was used during in vitro ruminal fermentation (Hansen et al., 2012; Leng et al., 2012a; Pereira et al., 2014; Saenab et al., 2018; Teoh et al., 2019). Biochar has been used as a feed additive to build microbial habitats and to alter biofilm activity in the rumen (Leng, 2014). Also, BC can increase the efficiency of microbial growth (Leng et al., 2012b, 2012c).
The degree of acidity (pH) of the ruminal fluid indicates the fermentation process in the rumen. The addition of BC and LS produced an average rumen pH of 6.9. The pH value was in the normal range of ruminal pH of 5.5–7.5 (Franzolin et al., 2010) and so did not interfere with microbial activity in the rumen, supported the growth of ruminal microbes, and produced VFAs and NH3. Although the pH of BC and LS were 10 and 3.75, respectively, they did not harm total gas production because the buffer solution added to the ruminal fluid was able to maintain the ruminal fermentation pH. The addition of 1–4% DM of BC had no negative effect on ruminal fermentation in either total gas production or the rate of gas production. Biochar supplementation in this experiment did not reduce CH4 in the rumen. As a greenhouse gas, CH4 contributes to global warming (Eisler et al., 2014) and also represents a certain level of energy loss from livestock (Jayanegara et al., 2018). A previous study by Hansen et al. (2012) which tested four types of BC added by 9% DM showed a reduction in CH4 emissions of 11–17%. Leng et al. (2012a) added 1% rice husk-based BC (DM) to a mixture of cassava and urea feed and showed a decrease in CH4 of 12%. Saenab et al. (2018) showed a decrease in CH4 when 0.3 mg/ml BC from cashew nut shells was added. The differing results of this experiment to other published reports may be due to differences in the biomass source of BC affecting BC properties such as pore size, adsorptive potential, added into the rumen fermentation, feed composition that may give different effect on methane production (Teoh et al., 2019). In addition, the level of BC addition affected methane reduction in a quadratic relationship, as reported by Saleem et al. (2018).
Ammonia (N-NH3) is the main source of nitrogen for microbial protein synthesis in the rumen and so concentration of ammonia in the rumen is a point for consideration. Ammonia is also the end product of protein degradation of ration by ruminal microbes (Suharlina et al., 2016). The concentration of ammonia therefore represents the degree of degradation of protein by rumen microbes (Sujarnoko et al., 2020). High concentration of ammonia allows increased microbial protein synthesis in the ruminal system because ammonia is the main precursor in the formation of microbial cells. There is no effect on ammonia concentration from the addition of BC or LS at different levels. The BC or LS addition may not inhibit bacterial growth or activity in the deamination process in the ruminal fluid, and this is in line with previous research which stated that there was no influence of BC addition on the concentration of NH3 in ruminal fluid in vitro (Pereira et al., 2014). In addition, NH3 ruminal concentration is influenced by the crude protein quality of the incubated substrate. Difference in the quality and number of different types of soluble protein, quickly degraded, moderately degraded, slowly degraded, and undegradable protein fractions in the substrate often affect the degree and rate of NH3 production in the rumen (Jayanegara et al., 2016).
Volatile fatty acids (VFA) are the final product of fermentation and are the main source of energy for ruminants and of carbon for the formation of microbial protein. The amount of VFAs indicates the fermentability of rations by ruminal microbes. The production of VFAs in the ruminal fluid can be used as a measure of ration fermentability. In this study, the addition of BC had the tendency to increase total VFAs compared to the BC0 treatment. Various effects of BC addition on total VFAs or individual VFAs have been reported. Some BCs create no response in total VFA production (McFarlane et al. 2017; Cabeza et al., 2018; Teoh et al. 2019) while others cause a significant increase of total VFAs (Pereira et al., 2014; Saenab et al., 2018; Saleem et al. 2018). Production of VFAs is consistent with an increase in the molar proportion of acetate, indicating an increase in fiber digestion. In contrast, there was a decrease in the ratio of butyrate, isobutyrate, valerate, and isovalerate, isobutyrate, and isovalerate being the products of branched amino acid degradation. Biochar addition significantly decreased the proportion of molar butyrate in the ruminal fluid starting from BC2, BC3, and BC4, however, BC1 did not significantly decrease the molar proportion of butyrate compared to the control (BC0). Likewise, the molar proportions of isobutyrate and isovalerate showed a decrease starting from BC1. Isobutyrate and isovalerate are products of degradation of branched amino acids. Reduction of isoacids by biochar indicates that there may be an inhibition of some protein degradation to branched amino acids. However, the inhibition might occur partly and the degradation of amino acids to ammonia didnot get affected since the ammonia production in the rumen was not significanly different among treatments. The mechanism of biochar that reduces certain proteolytic bacteria is unknown. The reduction of protein degradation in the rumen may result in a higher level of by pass protein entering the duodenum. Further in vivo research on BC addition and bypass protein is warranted. The addition of LS significantly (p < 0.05) increased total VFAs, acetate, and the ratio of acetate to propionate. The addition of LS had the tendency (P < 0.1) to lower butyrate and valerate amounts. Liquid smoke addition significantly decreased the molar proportion of isobutyrate and isovalerate in the ruminal fluid starting from LS1. A similar effect of BC and LS additions in reducing molar proportion of isobutyrate and isovalerate indicated an inhibition of protein degradation to isoacids. The mechanism of isoacid reduction of LS was different from that of BC. Liquid smoke contains phenolic compounds (Table 1) which could bind protein in feed and in microbes (Jayanegara et al., 2019) and therefore might increase bypass protein. Acetic acid is minimally used in the liver, and is oxidized to produce ATP in most of the body. Another important use of acetate for lipid synthesis is as the main source of acetyl CoA. Higher ratio of acetate:propionate has some effect on an animal’s efficiency, for example, higher acetate:propionate ratio appears to result in higher butterfat than milk yield in dairy animals (i.e. increased milk fat at the cost of milk yield).
Meanwhile, the proportion of propionate was not influenced by BC or LS addition. Pereira et al. (2014) reported that decreasing proportion of propionate had a relationship with total VFA production. Propionate development, on the other hand, implies a net incorporation of dihydrogen. Therefore, propionate competes in rumen fermentation with CH4 as a hydrogen drain, while acetate and butyrate formation release hydrogen that can be used by methanogens to reduce CO2 to CH4 (Janssen, 2010). Dihydrogen concentration in the rumen is influenced by factors influencing the growth of methanogens and the rate of feed fermentation, which in turn regulate the balance between pathways generating and integrating metabolic hydrogen, deciding the production of methane and the profile of VFAs (Ungerfeld, 2020). Biochar supplementation can increase the efficiency of energy use in ruminants. Acetate, propionate, and butyrate are the main products in ruminal fermentation processes, while VFAs are an important part of the energy for ruminants (Li et al., 2013).
Biochar addition significantly and progressively decreased IVRDMD and IVROMD compared to the control from BC2, BC3 and BC4; however, BC1 did not significantly decrease IVRDMD and IVROMD compared to the control (BC0). This is different from a study by Saenab et al. (2018) which added BC at different levels that did not significantly influence IVDMD and IVOMD compared to the control. In addition, different BC sources can give different ruminal fermentation responses, due to differences in structure and composition of basic ingredients, as well as the effectiveness of BC as a feed additive due to changes in feed digestion in the rumen (McFarlane et al., 2017). The addition of LS did not influence IVRDMD and IVROMD. This is in line with Saenab et al. (2018) who found that the supplementation of LS from cashew-nut shells did not influence feed degradation or gas production. The total phenol content in LS was 3.12%, and the supplementation of LS had no negative effect on the total values of gas production, the rate of gas production, IVRDMD, or IVROMD. Jayanegara (2009) reported that phenol concentrations of more than 5% can suppress livestock performance, so their use in rations should be below this level. Furthermore, phenolic compounds can interact with protein (Kondo et al., 2014) in cell walls or membranes and various pathogenic microbial enzymes and so can destroy microbes.
Increasing the level of BC and LS additions caused a decrease in the number of protozoa (about 6–7%). The decrease in the number of protozoa might contribute to the decrease in total bacterial counts and thus contribute to a decrease in IVRDMD and IVROMD (Newbold et al., 2015). Protozoa play a role in the ruminal fermentation process because they can reduce the main components of feed. One of the ciliated protozoa that has an important role in the rumen is Diploplastron affine, which can digest cellulose and carbohydrates from grains (Wereszka and Michałowski, 2012). Also, the holotrich protozoa, although in small amounts, also produce enzymes that are responsible for the degradation of cellulose and hemicellulose. Mosoni et al. (2011) suggest that a decrease in the protozoan population will harm fiber digestion, which is the main function of the rumen. Also, BC addition significantly decreased the bacterial population in the rumen from BC2, BC3, and BC4, however, BC1 treatment did not significantly decrease the total bacteria compared to the control (BC0). This is differs from a study by Teoh et al. (2019) which added 800 mg/d biochar (7.2% DM) and found that this did not significantly influence the total bacteria in the rumen, but suppressed several bacterial species, such as Methanomethylophilaceae, with a decrease of 19.8 times population, and Lactobacillus spp., with 31.7 times decrease in population. Rumen protozoa have a symbiotic relationship with methanogens involved in the transfer of hydrogen to reduce carbon dioxide to methane (Machmüller et al., 2003). When protozoa are reduced, these symbiotic methanogens would also reduce. In this experiment, however, the reduced methanogens did not decrease methane production in the rumen. The same result was recorded by Hegarty et al. (2008), who found that protozoa did not have a significant impact on the production of rumen methane when investigated in chemically defaunated, birth-defaunated, and faunated lambs. In addition, the release of methane without changes in protozoa numbers suggests that methanogenesis is often regulated by other non-protozoa mechanisms (Guyader et al., 2014).
CONCLUSION
The lowest level of biochar (0.1 mg/ml) and the lowest level of liquid smoke (0.25 μl/ml) from cacao pod husk addition to in vitro rumen fermentation tended to increase and significantly increased total VFAs, respectively. At their lowest level, both biochar and liquid smoke shifted VFA composition, reduced part-degradation of protein to branched amino acids and partly defaunated rumen microbes without affecting IVRDMD and IVROMD.
ACKNOWLEDGEMENT
This study was supported by the Indonesian Agency for Agricultural Research and Development, Ministry of Agricultural, Republic of Indonesia.
AUTHORS CONTRIBUTION
NQ conducted the experiment, performed data analysis, and wrote the article draft; EW checked data analysis and revised the article draft; YR and IGP supervised the experiment; AJ designed the experiment, checked data analysis, and revised the article draft.
CONFLICT OF INTEREST
All authors declare that there is no conflict of interest.
References