Advances in Animal and Veterinary Sciences
Review Article
Emerging Role of Regulatory T Cells in Heterologous Infections
Shalini Sharma1*, Naveen Kumar2
1Department of Veterinary Physiology and Biochemistry, Lala Lajpat Rai University of Veterinary and Animal Sciences, Hisar, Haryana 125004, India; 2Virology Laboratory, Division of Animal Health, Central Institute for Research on Goats, Indian Council of Agricultural Research, Makhdoom, P.O.-Farah, Mathura, UP-281122, India.
Abstract | Prior immunity can result in complete protection against a homologous agent. In the real world, however, each individual experiences multiple infections. Immune memory responses that are generated in an individual as a consequence of an initial or primary infection has been convincingly shown to influence the immune response and the course of infection of subsequent, unrelated pathogen challenge, by a process known as heterologous immunity. The fact that the host’s history of previous infections cannot be altered warrants our current understanding of the cellular mediators involved and the roles they play in regulating heterologous immunity. Several cellular mediators such as CD4, CD8T cells and various innate immune cells participate in mediating heterologous immunity. Regulatory T cells are an important subset of CD4T cells and here in this review we attempt to discuss our current understanding on the role of regulatory T cells (Tregs) in heterologous immune responses.
Keywords | Regulatory T cells, Heterologous infection
Editor | Ruchi Tiwari, College of Veterinary Sciences, Department of Veterinary Microbiology and Immunology Uttar Pradesh Pandit Deen Dayal Upadhayay Pashu Chikitsa, Vigyan Vishvidhyalaya Evum Go-Anusandhan Sansthan (DUVASU), Mathura (U.P.) – 281001, India.
Special Issue| 2 (2015) “Reviews on Trends and Advances in Safeguarding Terrestrial /Aquatic Animal Health and Production”
Received | January 12, 2015; Revised | February 05, 2015; Accepted | February 07, 2015; Published | February 12, 2015
*Correspondence | Shalini Sharma, Lala Lajpat Rai University of Veterinary and Animal Sciences, Hisar, Haryana, India; Email: [email protected]
Citation | Sharma S, Kumar N (2015). Emerging role of regulatory T cells in heterologous infections. Adv. Anim. Vet. Sci. 3(2s): 25-31.
DOI | http://dx.doi.org/10.14737/journal.aavs/2015/3.2s.25.31
ISSN (Online) | 2307-8316; ISSN (Print) | 2309-3331
Copyright © 2015 Sharma et al. This is an open access article distributed under the Creative Commons Attribution License, which permits unrestricted use, distribution, and reproduction in any medium, provided the original work is properly cited.
HETEROLOGOUS IMMUNITY
A very important and distinguishable feature of adaptive immunity is the generation of memory responses (Farber, 2005). Immune responses generated against a prior infectious agent can alter the immune response and the infection pattern of subsequent, unrelated pathogens by a process commonly defined as heterologous immunity (Martin, 2014; Sharma and Thomas, 2014). The outcome of heterologous infection can vary from good that is providing immunity to bad meaning resulting in a net immunopathology. The sequence of infections in heterologous infections may vary for example the infections can occur concurrently or sequentially. In a setting of infections with different strains of a similar pathogen or distinct pathogens are often classified as “coinfections” or super infections (Selin et al., 2011; Sharma and Thomas, 2014).
CD4T CELLS AS MEDIATORS OF HETEROLOGOUS IMMUNITY
The CD4 T cell plays a plays a very prominent role in providing protection against viral infections and are also involved in the development of memory B cells and CD8 T cells (Crotty and Ahmed, 2004; Konishi, 2013; Sun et al., 2014; Zinkernagel, 2002). CD8 T cells require CD4T cell help and this is important for primary and/or memory responses to some bacterial and viral infections (Bevan, 2004; Slifka, 2004; Wodarz, 2003). When we talk in context of heterologous immunity, CD4 T cells can play a protective role or it can worsen the course of infection resulting in immunopathology (Damjanovic et al., 2012). For instance the adoptive transfer of CD8 and CD4 subsets from LCMV immune mice into naive mice resulted in heterologous immunity upon subsequent infections with Pichinde virus or Vaccinia virus (Selin et al., 1998). But we still lack the literature on the role of CD4T cells especially in the settings of various types of heterologous infections.
CD4T cells have been shown to protect against heterologous infections in a bystander manner. For example a study with BCG strain of Mycobacterium bovis (a vaccine against tuberculosis) suggested that immunization of mice against the BCG strain of Mycobacterium bovis also protected the animals against challenge with Vaccinia virus (VV), belonging to family Poxviridae. CD4T cells mediated this protection through production of IFNγ.
When an exacerbated immune response is generated, the immune system has developed several mechanisms to regulate these excessive immune responses that may cause pathology and autoimmune disease. These regulative mechanisms include existence of regulatory T cells (Belkaid et al., 2002; Suvas et al., 2003), certain inhibitory interactions such as Tim3/Galectin 9 inhibitory interaction (Belkaid et al., 2002; Kuchroo et al., 2006; Kuchroo et al., 2008; Sharma et al., 2011; Su et al., 2011; Zhu et al., 2005), PD1-PDL1 axis (Rouse and Sehrawat, 2010), CTLA-4 and CD80/86 (Rouse and Sehrawat, 2010) interactions.
REGULATORY T CELLS
Tregs are an important subset of CD4 T cells (La Cava et al., 2006). The Tregs that develop in the thymus are known as naturally occurring Treg cells and the adaptive Tregs that are induced by various stimulations such as an antigen (Benoist and Mathis, 2012; French and Kinter, 2012; Robertson and Hasenkrug, 2006; Rouse et al., 2006). Naturally occurring CD4+CD25+ regulatory T cells have been shown to constitute 5–15% of peripheral CD4+ T in both mice and humans (Sakaguchi, 2003). A large body of literature suggests that the severity of many immune inflammatory reactions are regulated or controlled by Tregs (Suvas and Rouse, 2006). The Tregs acts to limit or suppress the immune response generated against a pathogen (Suvas et al., 2004). We need this Treg mediated suppression to prevent the collateral tissue damage that might occur due to the excessive immune response generated against the pathogen (Sehrawat et al., 2008). Thus Tregs greatly influence the magnitude of a T cell mediated immune response to an acute viral infection (Sharma et al., 2014; Suvas et al., 2003) particularly those that express FoxP3.
Infections or challenge with the pathogens may signal Treg expansion and this Treg expansion could further influence the magnitude and also the pattern of the immune response. Thus in such cases immune responses against a pathogen and responses to vaccines are elevated if the Treg response is modulated (Rouse and Suvas, 2004; Sehrawat et al., 2008; Suvas et al., 2004).
The magnitude of the Treg response in different individuals may also explain in some circumstances the differential outcome of infection with chronic infection like hepatitis (Rouse and Sehrawat, 2010). In case of persistent infections where there is huge tissue damage, the Treg response can improve the outcome and diminish the pathology (Sehrawat and Rouse, 2011). In many studies the Treg response to pathogens is assumed to consist mainly or predominately of antigen specific Tregs (Bedoya et al., 2013), but there are some examples to cite where the involvement of Tregs is not antigen speific (Maizels et al., 2004; Telford et al., 1998; Bedoya et al., 2013) and a recent study has shown a possible mechanism of generation of non-antigen specific Tregs (Sharma et al., 2014).
REGULATORY T CELLS IN HETEROLOGOUS INFECTIONS
Here we discuss the Treg cells expanded during past infections that can potentially modulate the characteristics of effector T-cell responses and immunopathology during subsequent heterologous infections. The influence of natural Treg cells may favourably affect the outcome or can be harmful to the host (Page et al., 2006). However, the outcome of an infection (viral) may be affected by several other factors such as the stage of infection (Figure 1A, 1B and 1C), dose of the pathogen and genotype and immunological status of the host and also the presence of various other diseases and infections (Belkaid and Rouse, 2005).
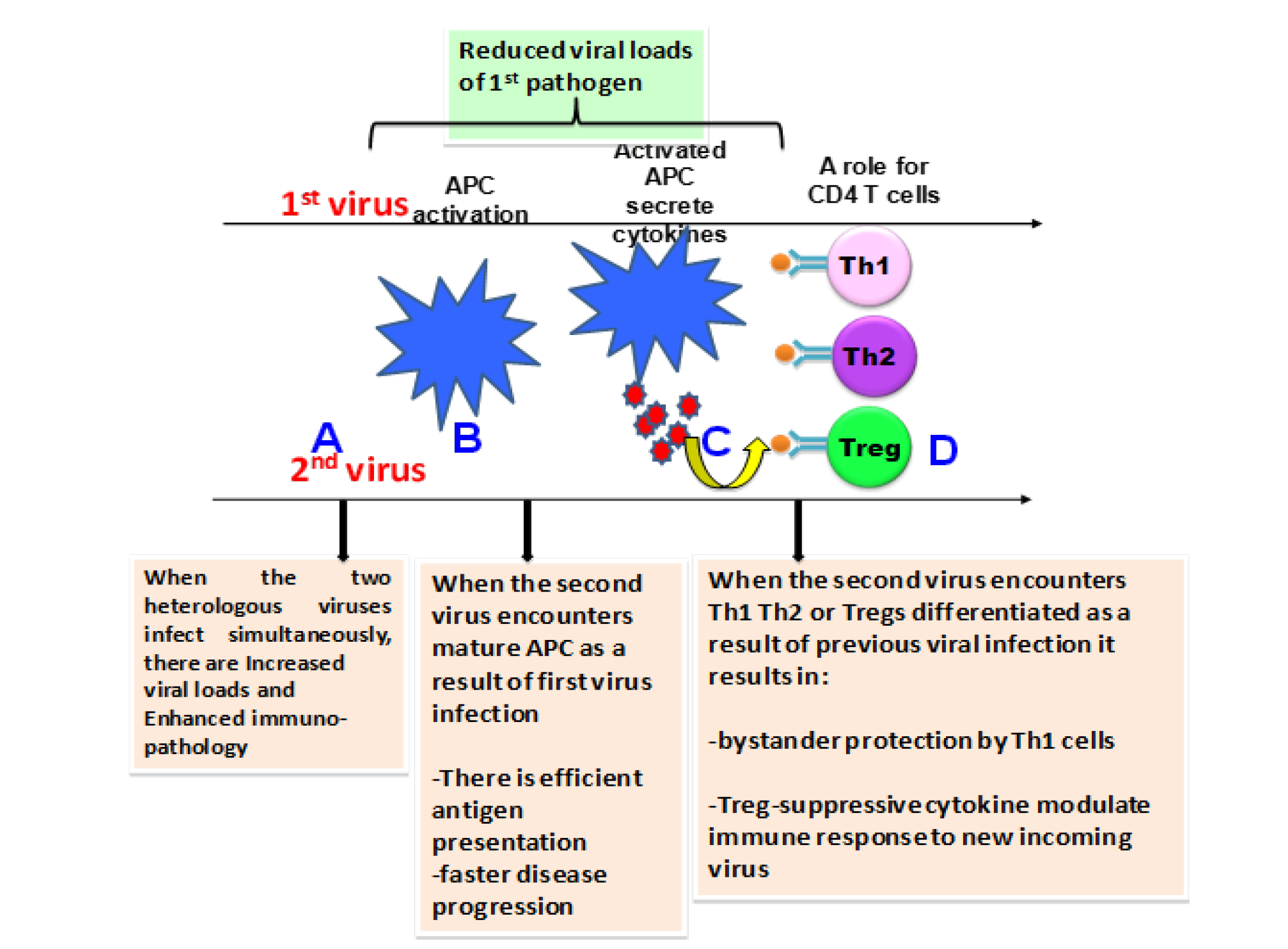
Figure 1: Outcome of infection following heterologous viral infections is dependent on the stages of ongoing immune response to a previously encountered pathogen
(A) If the infections with the viruses (here for example 2 virus system) occurs at the same time, the outcome could be enhanced immunopathology possibly due to the immune responses to both the viruses reaching the peak at the same time. (B) Prior infection can result in activation of APC and the new incoming infection at this stage encounters mature APC, efficient antigen presentation and faster disease progression. Also the new incoming pathogen creates a strong antiviral state that might result in reduced viral loads of first pathogen. (C) Upon activation APC’s secrete cytokines that results in T helper subset differentiation and the incoming pathogen at this stage encounters a polarized immune response. Encounter with Th1 type of immune response can provide bystander protection or enhanced imunopathology whereas T regs (D) can result in suppression of immune responses to incoming pathogen, which may be protective or pathogenic. The outcome of heterologous viral infections however, depends on the type and sequence of viruses.
Although the role of Tregs has not been extensively evaluated in co-infection models, however several studies in hetrologous infections suggests that Tregs induced during one infection (Figure 1D) can lead to suppression of bystander responses (Page et al., 2006). The First evidence that depleting Tregs prior to viral infection enhances CD8T cell responses to subsequent viral infection came from the studies by Suvas et al. (2003). Their study demonstrated that depletion of Tregs resulted in increased activation and proliferation of Herpes Simplex virus (HSV-1) specific CD8T cells and this effect was observed both in the acute and memory phases of the immune response. These findings were very important in terms of explaining the phenomenon of diminished immunity during a viral infection or other infectious agents and suggested the fact that vaccine responses can be improved by Treg manipulation. This study also suggested how viral infection might temporarily diminish immunity to other infectious agents and thus their application to vaccines. Thus, controlling suppressor effects of regulatory T cells at the time of vaccination could result in more effective immunity.
Later on it was shown that that Treg depletion via treatment with anti-CD25 mAb (PC61) significantly enhances CD8T cell responses to influenza A virus, vaccinia virus, and SV40-transformed cells induced by either direct priming or cross-priming (Haeryfar et al., 2005). Importantly treatment with PC61 did not enhance CD8T cell responses in the absence of CD4T cells suggesting that PC61 acts on a subset of CD4 T cells, and not on other cells that express CD25. Tregs thus selectively suppress the responses to immuno-dominant CD8 T cell epitopes.
Treg cells generated during an acute infection can influence the magnitude and quality of effector T-cell responses and their ability to contribute to lung pathology during a subsequent heterologous virus infection as is shown for Influenza A virus infection (Brincks et al., 2013). Accordingly, following challenge with heterosubtypic IAV infection, antigen-specific IAV-induced Treg cells have been reported to attenuate subsequent T cell responses and decrease pathology during a secondary heterologous IAV challenge (Brincks et al., 2013).
The presence of Tregs has been convincingly shown to influence viral clearance and immunopathology in the setting of persistent viral infections both in human and animal models (Reuter et al., 2012; Tseng et al., 2012). In line with this using a heterologous infection model of persistent and non-persistent viral infections, it was shown for the first time that virus-expanded Treg cells could attenuate immune responses and influence induction of lung pathology during a subsequent unrelated non persistent virus infection (Kraft et al., 2013).
Tregs are usually known to result in a diminished immunopathology as has been shown in autoimmune diseases such as inflammatory bowel or celiac disease (Chen et al., 2003), and in some viral infections, such as respiratory syncytial virus (RSV) (Fulton et al., 2010; Ruckwardt et al., 2009) and IAV (Bedoya et al., 2013; Brincks et al., 2013). And when these Tregs were depleted by PC61 treatment prior to acute viral infections such as corneal HSV-1, neonatal HSV-1, or i.n. RSV, this results in enhanced immunopathology due to increased virus-specific T-cell responses (Fulton et al., 2010; Ruckwardt et al., 2009; Suvas et al., 2004). However in contrast to these above mentioned studies, in the heterologous infection model of LCMV and IAV infection, depletion of Treg cells in IAV-immune mice prior to LCMV infection resulted in decreased lung pathology, with no differences in viral titters and significantly decreased LCMV-specific CD8+ T-cell responses in the spleen but not the mLN.
It was then suggested that this was due to the delay in effector T cells trafficking out of the lymph node in the absence of Treg cells in both the LCMV-infected Influenza A virus (IAV)-immune and the naive mice, which is consistent with previous reports demonstrating that Treg cells play a role in controlling egress of effector T cells from the lymph node (Lund et al., 2008). The important point to note here is that depletion of the IAV-expanded Treg cells during LCMV infection did not significantly enhance virus-specific T-cell responses (Haeryfar et al., 2005; Ruckwardt et al., 2009), which is in contrast to the findings with Treg depletion via PC61 treatment during acute RSV or IAV infection.
Another explanation for the significant reduction in the severity of lung pathology following depletion of Tregs appeared to be mediated by over-activation and subsequent partial exhaustion of the LCMV-specific CD8+ T-cell response in LCMV-infected, Treg cell-depleted, IAV-immune mice.
In a mouse model of respiratory viral infection it has been previously shown that influenza (flu)-immune compare to naïve mice infected with Lymphocytic choriomeningitis virus (LCMV) have enhanced viral load, severe lung pathology and an altered cytokine profile. In a study it was found that more CD4+Foxp3+ regulatory T (Treg) cells were present in lungs of these flu-immune mice compared to naïve or LCMV-immune mice thus raising the possibility that modulation in the normal balance of Treg and effector T cell responses might be contributing to these altered responses in flu-immune mice infected with LCMV. FLu-immune mice had altered kinetics compared to naïve mice infected with LCMV.
In this heterologous infection model of IAV and LCMV, Treg cells followed the same kinetics as CD4+ and CD8+ T cells in the mLN of acutely LCMV-infected mice that peaked at day 3 and declined at day 7, in flu-immune mice there was a persistent Treg population at higher levels, until day 9 after LCMV infection. The presence of increased Treg cells in flu-immune lungs and an altered Treg cell kinetics in subsequent heterologous LCMV respiratory infections results in increased viral load and enhanced pro-inflammatory cytokine and chemokine levels in the lungs and subsequent immunopathology.
Thus, in conclusion, an individual’s history of infection and specific sequence of infection can alter Treg cell populations, resulting in greatly altered disease outcome during subsequent new infections. For a better understanding of the role of Tregs in co-infection systems, our limited literature warrants more detailed studies with various hetrologous infection models in future.
CONFLICT OF INTEREST
Authors declare no conflict of interest.
REFERENCES