Advances in Animal and Veterinary Sciences
Research Article
Prophylactic Role of Moringa oleifera Leaves’ Extract against Lead Toxicity in Rabbits
Nancy B. Mohamed1,2, Amira H. Mohamed2, Nashwa A. Abu-Aita2, Soad M. Nasr3*, Somia A. Nassar3,4, Kawkab A. Ahmed5
1Directorate of Veterinary Medicine, 3 Teraet El-Zomor St., Nasr El Din, El-Haram, Giza, Egypt; 2Department of Clinical Pathology, Faculty of Veterinary Medicine, Cairo University, Giza 12211, Egypt; 3Department of Parasitology & Animal Diseases, National Research Centre, 33 Bohouth St., Dokki, Giza 12622, Egypt; 4Department of Medical Laboratory Sciences, College of Applied Medical Sciences, Prince Sattam Bin Abdulaziz University, Al-Kharj, Saudi Arabia; 5Department of Pathology, Faculty of Veterinary Medicine, Cairo University, Giza 12211, Egypt.
Abstract | An experiment was carried out to evaluate Moringa oleifera leaves ethanol extract (MOLEE) as a prophylactic treatment of lead acetate-induced toxicity in rabbits. Serum biochemical parameters, oxidant/antioxidant markers, and histopathology of liver and kidney were determined. The effect of long-term MOLEE treatment was also evaluated. Forty-eight male New Zealand white rabbits were randomly divided into four equal groups: normal control group; MOLEE-treated group, administered MOLEE (orally, 400 mg kg-1day-1) for successive 12 weeks; lead-intoxicated group, administered lead acetate (orally, 40 mg kg-1day-1) for successive 8 weeks; and MOLEE-lead-intoxicated group, administered MOLEE (orally, 400 mg kg-1day-1) for successive 12 weeks simultaneously with lead acetate (orally, 40 mg kg-1day-1) for successive 8 weeks. The results revealed that MOLEE treatment significantly (P<0.05) increased body gain and impacted positively on lipid profile, glucose, liver, and renal functions. On the other hand, administration of lead acetate caused marked (P<0.05) drop in body weight gain and elevated the activities of AST, ALT, and ALP and the levels of urea and creatinine, lipid profile, and lipid peroxidation as well as histopathological changes in the liver and kidneys. After 4 weeks of treatment, MOLEE-lead-intoxicated rabbits showed slight cytoplasmic vacuolization of hepatocytes and slight congestion of glomerular tuft, while vacuolation of epithelial lining renal tubules was noticed in kidneys of the lead-intoxicated group. In conclusion, prophylactic use of MOLEE has the ability to mitigate the alterations of serum biochemical parameters and histopathological architecture of liver and kidneys caused by lead acetate after 4 weeks from the start of treatment. Results of the present experiment revealed that Moringa oleifera has a curing effect on lead toxicity; nevertheless, further studies are required to determine the safety of prolonged use of Moringa oleifera.
Keywords | Lead acetate, Moringa oleifera, Serum biochemistry, Histopathology, Rabbits.
Received | July 14, 2020; Accepted | July 22, 2020; Published | September 01, 2020
*Correspondence | Soad M. Nasr, Department of Parasitology and Animal Diseases, Veterinary Research Division, National Research Centre, 33 Bohouth Street, Dokki, P.O. Box 12622, Giza, Egypt; Email: [email protected]
Citation | Mohamed NB, Mohamed AH, Abu-Aita NA, Nasr SM, Nassar SA, Ahmed KA (2020). Prophylactic role of moringa oleifera leaves’ extract against lead toxicity in rabbits. Adv. Anim. Vet. Sci. 8(11): 1129-1141.
DOI | http://dx.doi.org/10.17582/journal.aavs/2020/8.11.1129.1141
ISSN (Online) | 2307-8316; ISSN (Print) | 2309-3331
Copyright © 2020 Mohamed et al. This is an open access article distributed under the Creative Commons Attribution License, which permits unrestricted use, distribution, and reproduction in any medium, provided the original work is properly cited.
INTRODUCTION
A lot of studies proved that exposure to different types of environmental pollutants is strongly associated with renal and hepatic tissue damage (Gao et al., 2015). Lead, a notably dangerous pollutant, proved to be hepato- and nephrotoxic to humans and the majority of animal species (Soliman et al., 2015). In postmortem studies conducted on humans exposed to lead, the highest prevalence of lead in the soft tissues was found in the liver (33%) followed by the kidneys (Dadpour et al., 2016). The mechanisms causing lead toxicity involve disorganized oxidative balance leading to increase in the production of reactive oxygen species (ROS) (Mitra et al., 2017). Lead causes neurological, circulatory, gastrointestinal, immunological, reproductive, histopathological, and histochemical effects in animals and humans (Carocci et al., 2016).
Moringa oleifera Lam. (kingdom: Plantae, order: Brassicales, family: Moringaceae, genus: Moringa, species: Moringa oleifera) is one of the most important plants widely cultivated all over the world and is native to the Indian subcontinent. It is a fast-growing, drought-resistant drumstick tree (Raja et al., 2016). Besides its uses in water purification and its nutritional advantages, this plant is greatly esteemed for its medicinal value. Many medicinal attributes of Moringa oleifera have been ascribed to different parts of this highly marveled tree. All the components of the tree, i.e., leaves, bark, root, flowers, fruit, gum, pods, seed, and seed oil, have been exploited for treating different complaints in traditional medicinal systems as well as nutrition (Gopalakrishnan et al., 2016; Farid and Hegazy, 2019; Atta et al., 2019; Abdel-Rahman et al., 2019). In our previous study, M. oleifera leaves ethanol extract, as an adjunct treatment, had the potential to prevent the alterations caused by lead acetate-induced oxidative injuries and restore normal hepatic and renal functions (Mohamed et al., 2020).
Therefore, this study was performed to assess Moringa oleifera leaves ethanol extract (MOLEE) as a potential prophylactic treatment for lead acetate-induced hepatic and renal toxicity in rabbits. Serum biochemical parameters, oxidant/antioxidant markers, and histopathology of liver and kidney were determined. The effect of long-term Moringa oleifera treatment was also assessed.
MATERIALS AND METHODS
Ethics Statement
This experiment was performed according to the guidelines of the Institutional Animal Care and Use Committee (IACUC), Cairo University, Approval Protocol No. CU-II-F-94-18, in 11/2018.
Plant Used and Preparation of MOLEE
Moringa oleifera (family: Moringaceae) was obtained from the Egyptian Scientific Society for Moringa at National Research Centre, Dokki, Giza, where the plant was identified by Professor Dr. Aboelfetoh M. Abdalla and a voucher sample number (MO19) was kept in the Horticulture and Crops Technology Department. MOLEE was prepared according to the method of Mohamed et al. (2020). The crude extract was stored at –4°C until used.
Lead Acetate
Lead acetate trihydrate was purchased from Sigma-Aldrich (Merck), Germany. It was dissolved in distilled water at a concentration of 40 mg mL-1 for oral administration.
Animal Used and Experimental Design
This experiment was performed at the Experimental Rabbit Unit of Lab Animal House, National Research Centre, Egypt. Male New Zealand white rabbits (weight: 1-1.5 kg; age: 4–6 weeks) were purchased from a farm belonging to Animal Production Institute, Ministry of Agriculture, Dokki, Giza. Rabbits were housed under hygienic conditions in separate stainless steel cages at a controlled temperature (23±2 C), with light/dark cycle (12 h) and relative humidity (50%–60%). All rabbits were fed a balanced commercial growing rabbit pelleted diet, with ad libitum fresh tap water.
Forty-eight rabbits were randomly assigned to four equal groups. The first group was kept as normal control. The second one (MOLEE-treated) was orally administered MOLEE at a dose of 400 mg kg-1day-1 for successive 12 weeks. The third group (lead-intoxicated) was orally administered lead acetate at a concentration of 40 mg kg-1day-1 for successive 8 weeks (5 days/week). The fourth group (MOLEE-lead-intoxicated) was orally given MOLEE at a dose of 400 mg kg-1day-1 for successive 12 weeks simultaneously with lead acetate orally at a concentration of 40 mg kg-1day-1 for successive 8 weeks (5 days/week). All experimental animals were daily monitored for abnormal clinical signs, and mortalities were recorded. Body weight was recorded before the start of the experiment (zero time) and every week thereafter. Body weight gain was calculated by deducting the weight of the animal at start of the experiment (zero time) from the body weight in the 4th, 8th, and 12th week of the experiment.
Sampling
In the 4th, 8th, and 12th week of the experiment, blood samples were collected from the ear-vein puncture from 5 rabbits of each group in the early morning before feeding. Each blood sample was put into a plain vacutainer tube, left to clot then centrifuged at 3000 rpm for 15 min and serum was separated. The serum samples were stored at −20 OC for further biochemical analysis. After collection of the blood samples, three animals from each group were sacrificed, and specimens were obtained from liver and kidneys for histopathological examination as well as assessment of oxidant/antioxidant markers in liver tissue.
Serum Biochemical Analysis
The following serum biochemical parameters were measured using the methods indicated in parentheses: activities of alanine aminotransferase (ALT) and aspartate aminotransferase (AST) (Reitman & Frankel, 1957); alkaline phosphatase (Roy, 1970); glucose (Trinder, 1969); creatinine (Houot, 1985); urea (Patton & Crouch, 1977); total cholesterol (Richmond, 1973); triglycerides (Fossati & Prencipe, 1982); high-density lipoprotein cholesterol (HDL-C) (Albers et al., 1978); low-density lipoprotein cholesterol (LDL-C) (Friedewald equation, Friedewald et al., 1972); total proteins (Gornal et al., 1949); and albumin (Doumas et al., 1971). Total globulins were calculated by subtracting the obtained value of albumin from the total proteins. All parameters were determined using a spectrophotometer (T80 UV/VIS, PG Instrument Ltd., UK) with commercial test kits from Biodiagnostic Co, Egypt.
Oxidant/Antioxidant Analysis in Liver Tissue and Serum
Liver homogenate were prepared according to the method of Mohamed et al. (2020). Briefly, a portion of liver tissue was weighed, washed, and homogenized with ice-cold normal saline (10% w/v) using a homogenizer (Witeg, Germany). The homogenate was then centrifuged at 4,000 rpm for 5 min at 4°C. The supernatant was collected and stored at -80°C until used for determination of the levels of reduced glutathione (GSH) (Ellman, 1959) and lipid peroxidation by-products such as malondialdehyde (MDA) (Ohkawa et al., 1979). Nitric oxide (NO) was determined in serum (Rajarman et al., 1998).
Histopathological Examinations
Specimens from the liver and kidney were collected from all experimental groups, preserved in neutral buffered formalin 10%. Specimens were then washed, dried, cleared, and immersed in paraffin. Paraffin blocks were sliced into 5-micron-thick sections and stained with hematoxylin and eosin (H&E) (Suvarna et al., 2019).
Statistical Analysis
The data are expressed as mean ± standard division (mean ± SD). Statistical significance between groups was assessed using the one-way analysis of variance (ANOVA). Then, Duncan’s multiple range test (Petrie & Watson, 2013) was performed using SPSS software version 17 (SPSS Inc., Chicago, IL, USA). P values<0.05 were considered significant.
RESULTS
Clinical Signs
Rabbits exposed to lead intoxication showed depression, loss of appetite, lethargy, and easy hair falling, and one animal died during the 8th week of treatment. In the other groups, no mortalities or morbidities occurred during this experiment.
Body Weight Gain
In the MOLEE-treated group, the body weight gain markedly (P<0.05) increased compared to the other groups during the experiment periods (in the 4th, 8th, and 12th week). On the other hand, the body weight gain in the lead-intoxicated group markedly (P<0.05) decreased compared to MOLEE-lead-intoxicated group (Figure 1).
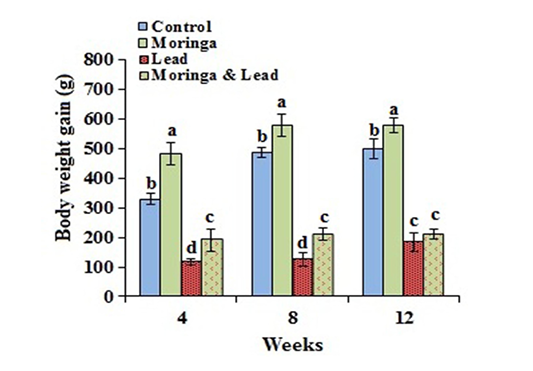
Figure 1: Body weight gain of male rabbits exposed to lead acetate and/or Moringa oleifera ethanol extract (mean SD, N = 5). Means with different letters (a, b, c, d) in the same period are significant at P<0.05.
Serum Biochemical Findings
These results are illustrated in Tables 1-2 and Figures 2-3.
After 4 weeks of treatment, no significant difference in the activity of ALT and AST was seen between all groups. After 8 weeks of treatment, the activity of ALT and AST significantly (P<0.05) elevated in both MOLEE-treated and lead-intoxicated groups compared to the other groups. After 12 weeks of treatment, ALT activity markedly (P<0.05) increased in the MOLEE-treated group compared to the other groups, while AST values significantly (P<0.05) increased in the MOLEE-lead-intoxicated rabbits than the other groups. After 4 weeks of treatment, the activity of ALP markedly (P<0.05) elevated in the lead-intoxicated group compared to the others. However, after 8 and 12 weeks of treatment, ALP activity was markedly (P<0.05) decreased in the MOLEE-lead-intoxicated rabbits than that in the other groups (Figure 2).
In the 4th week of the experiment, serum glucose levels significantly (P<0.05) went up in the lead-intoxicated group but went down in both MOLEE-treated and MOLEE-lead-intoxicated groups compared to control ones. Moreover, glucose levels significantly (P<0.05) increased in both lead-intoxicated and MOLEE-lead-intoxicated groups in the 8th week compared to control and MOLEE-treated groups. After 12 weeks, the glucose levels significantly (P<0.05) increased in MOLEE-treated, lead-intoxicated, and MOLEE-lead-intoxicated groups compared to the control one (Figure 2).
In the 12th week posttreatment, total serum proteins and
globulins significantly (P<0.05) increased in the lead-intoxicated group compared to the other groups. On the other hand, serum albumin levels markedly (P<0.05) dropped 12 weeks after treatment in both lead-intoxicated and MOLEE-lead-intoxicated groups compared to control and MOLEE-treated groups (Table 1).
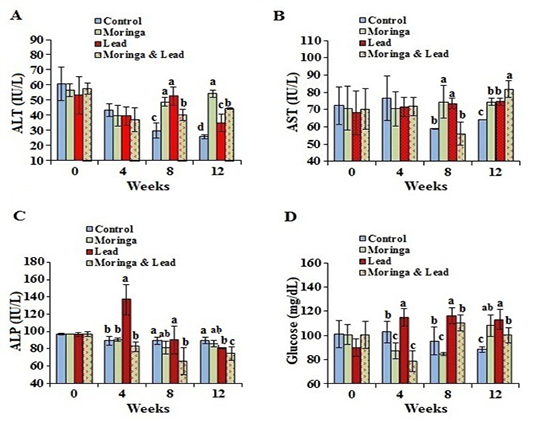
Figure 2: Serum enzymes activity and glucose of male rabbits exposed to lead acetate and/or Moringa oleifera ethanol extract. (A) alanine aminotransferase (ALT), (B) aspartate aminotransferase (AST), (C) alkaline phosphatase (ALP), (D) glucose concentrations (mean SD, N = 5). Means with different letters (a, b, c, d) in the same period are significant at P<0.05.
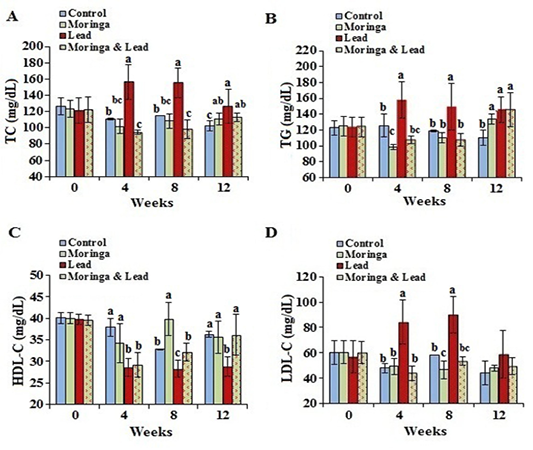
Figure 3: Serum lipid profile of male rabbits exposed to lead acetate and/or Moringa oleifera ethanol extract. (A) total cholesterol (TC), (B) triglycerides (TG), (C) high-density lipoprotein cholesterol (HDL-C), (D) low-density lipoprotein cholesterol (LDL-C) (mean SD, N = 5). Means with different letters (a, b, c) in the same period are significant at P<0.05.
In the 4th and 8th week posttreatment, the values of TC and TG showed significant (P<0.05) increase in the lead-intoxicated group compared to the other groups. Moreover, in the 12th week posttreatment, TC and TG significantly (P<0.05) increased in the MOLEE-treated, lead-intoxicated, and MOLEE-lead-intoxicated groups compared to control ones. In the 8th week, the values of HDL-C significantly (P<0.05) increased in the MOLEE-treated group compared to the other groups. However, HDL-C levels markedly (P<0.05) decreased in both lead-intoxicated and MOLEE-lead-intoxicated groups during the period of treatment. In the 4th and 8th week posttreatment, LDL-C levels significantly (P<0.05) increased in lead-intoxicated group compared to the other groups (Figure 3).
In the 8th week of experiment, blood serum urea and creatinine concentrations markedly (P<0.05) increased in the lead-intoxicated group compared to the others, while they significantly (P<0.05) increased after 12 weeks in both MOLEE-treated and lead-intoxicated groups compared to the others (Table 1).
Oxidant/Antioxidant Status
After 4 weeks of treatment, hepatic GSH levels significantly (P<0.05) decreased in the lead-intoxicated rabbits but markedly increased in the MOLEE-lead-intoxicated group compared to the other groups. After 8 weeks of treatment, GSH values significantly (P<0.05) decreased in the lead-intoxicated group compared to the others. No statistical difference was recorded between all groups after 12 weeks (Table 2).
Hepatic lipid peroxidation by-product (MDA) levels showed significant (P<0.05) increase in the 4th and 8th week in the lead-intoxicated group and in the 8th week in the MOLEE-treated group compared to other groups. After 12 weeks of treatment, MDA levels significantly (P<0.05) increased in both MOLEE-treated and MOLEE-lead-intoxicated groups compared to the others.
Serum NO levels displayed a significant (P<0.05) increase in lead-intoxicated group in the 4th and 8th week posttreatment compared to the other groups. However, after 12 weeks, NO levels markedly (P<0.05) increased in both MOLEE-treated and MOLEE-lead-intoxicated groups compared to the lead-intoxicated and control groups (Table 2).
Histopathological Findings
Microscopical examination of the liver sections of control rabbits showed normal histological structure of hepatic lobules (Figure 4A). The liver of MOLEE-treated rabbits (4 weeks) revealed no histopathological alterations (Figure 4B). On the other hand, liver of rabbits treated with
Table 1: Serum proteins profile, blood urea, and creatinine concentrations of male rabbits exposed to lead acetate and/or Moringa oleifera ethanol extract (MOLEE) (mean SD, N = 5).
Parameters | Normal control | MOLEE | Lead | Lead + MOLEE |
Total proteins (g/dL) | ||||
0 weeks | 6.17 ± 0.04 | 6.16 ± 0.04 | 6.17 ± 0.08 | 6.18 ± 0.06 |
4 weeks | 6.03 ± 0.25 | 6.08 ± 0.26 | 6.12 ± 0.51 | 6.12 ± 0.70 |
8 weeks |
6.20 ± 0.10ab |
6.47 ± 0.71a |
6.36 ± 0.59a |
5.65 ± 0.05b |
12 weeks |
6.27 ± 0.04c |
6.65 ± 0.35b |
7.32 ± 0.38a |
6.73 ± 0.04b |
Albumin (g/dL) | ||||
0 weeks | 3.43 ± 0.04 | 3.41 ± 0.02 | 3.41 ± 0.05 | 3.42 ± 0.03 |
4 weeks |
3.40 ± 0.12ab |
3.34 ± 0.11ab |
3.23 ± 0.18b |
3.52 ± 0.15a |
8 weeks |
2.70 ± 0.10b |
3.23 ± 0.27a |
3.43 ± 0.38a |
3.15 ± 0.15a |
12 weeks |
3.75 ± 0.25a |
3.81 ± 0.02a |
3.49 ± 0.12b |
3.43 ± 0.11b |
Total globulins (g/dL) | ||||
0 weeks | 2.75 ± 0.05 | 2.76 ± 0.04 | 2.77 ± 0.06 | 2.76 ± 0.07 |
4 weeks | 2.63 ± 0.15 | 2.74 ± 0.31 | 2.89 ± 0.58 | 2.60 ± 0.70 |
8 weeks |
3.50 ± 0.20a |
3.24 ± 0.48a |
2.69 ± 0.37b |
2.50 ± 0.20b |
12 weeks |
2.52 ± 0.24b |
2.85 ± 0.35b |
4.77 ± 1.11a |
3.27 ± 0.11b |
Urea (mg/dL) | ||||
0 weeks | 62.16 ± 3.44 | 62.16 ± 3.45 | 61.99 ± 3.43 | 62.16 ± 3.46 |
4 weeks | 49.87 ± 2.24 | 54.11± 5.24 | 54.64 ± 5.55 | 48.28 ± 7.29 |
8 weeks |
54.00 ± 10.00b |
45.37 ± 1.92c |
76.02 ± 3.38a |
49.58 ± 1.38bc |
12 weeks |
50.30 ± 3.70b |
70.73 ± 17.54a |
77.32 ± 3.41a |
49.52 ± 8.25b |
Creatinine (mg/dL) | ||||
0 weeks | 0.86 ± 0.04 | 0.84 ± 0.04 | 0.87 ± 0.06 | 0.84 ± 0.05 |
4 weeks | 0.77 ± 0.04 | 0.74 ± 0.09 | 0.75 ± 0.15 |
0.74 ± 0.15 |
8 weeks |
0.95 ± 0.05b |
0.96 ± 0.04b |
1.06 ± 0.09a |
0.75 ± 0.05c |
12 weeks |
0.95 ± 0.05c |
1.31 ± 0.02a |
1.11 ± 0.01b |
0.90 ± 0.10c |
Means with different letters in the same row are significantly different at P<0.05.
Table 2: Oxidant/antioxidant profile in tissue and serum of male rabbits exposed to lead acetate and/or Moringa oleifera ethanol extract (MOLEE) (mean SD, N = 5).
Parameters | Normal control | MOLEE | Lead | Lead + MOLEE |
Reduced glutathione (nmol/g Liver tissue) | ||||
4 weeks |
10.24 ± 0.15b |
10.30 ± 0.07b |
8.40 ± 0.82c |
12.72 ± 1.30a |
8 weeks |
10.32 ± 0.04a |
10.38 ± 0.29a |
8.00 ± 0.61c |
9.84 ± 0.09b |
12 weeks | 10.30 ± 0.07 | 9.02 ± 0.08 | 9.97 ± 0.41 | 10.36 ± 1.88 |
Lipid peroxidation by-products as malondialdehyde (µmol/g Liver tissue) | ||||
4 weeks |
0.53 ± 0.07b |
0.61 ± 0.14b |
0.94 ± 0.08a |
0.58 ± 0.08b |
8 weeks |
0.54 ± 0.08d |
1.52 ± 0.01a |
1.54 ± 0.04a |
1.24 ± 0.12b |
12 weeks |
0.54 ± 0.05d |
1.81 ± 0.01a |
0.92 ± 0.15c |
1.47 ± 0.22b |
Nitric oxide (µg/mL serum) | ||||
0 weeks | 4.30 ± 1.10 | 4.34 ± 1.10 |
4.36± 1.06 |
4.34 ± 1.07 |
4 weeks |
4.33 ± 1.07c |
5.02 ± 1.17c |
10.44 ± 2.41a |
7.40 ± 1.37b |
8 weeks |
4.05 ± 0.57c |
5.33 ± 0.84b |
7.70± 0.61a |
4.83 ± 1.06bc |
12 weeks |
4.07 ± 0.62d |
6.73 ± 0.55a |
5.10 ± 0.44c |
5.87 ± 0.66b |
Means with different letters in the same row are significantly different at P<0.05.
MOLEE (8 weeks) displayed focal hepatic necrosis associated with inflammatory cell infiltration (Figure 4C), cholangitis, and sinusoidal leukocytosis. Additionally, after 12 weeks of MOLEE treatment, examined sections revealed cytoplasmic vacuolization of hepatocytes, hyperplasia of biliary epithelium, and fibroplasia around the bile duct (Figure 4D).
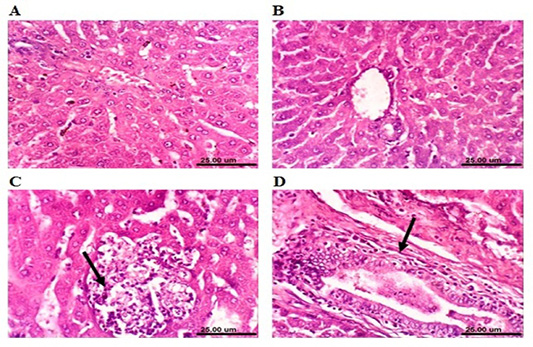
Figure 4: Photomicrograph sections from liver of a male rabbit. (A) Normal control group showing the normal histological structure of hepatic lobule. (B) Moringa oleifera leaf ethanol extract- (MOLEE-) treated group (4 weeks) showing no histopathological alterations. (C) Moringa oleifera extract-treated group (8 weeks) showing focal hepatic necrosis associated with inflammatory cell infiltration (arrow). (D) Moringa oleifera extract-treated group (12 weeks) showing hyperplasia of biliary epithelium and fibroplasia around the bile duct (arrow). (H&E, scale bar: 25 µm)
The liver of lead-intoxicated rabbits (4 weeks) revealed activation of Kupffer cells and cytoplasmic vacuolization of hepatocytes (Figure 5A). Examined sections from lead-intoxicated rabbits (8 weeks) showed focal hepatocellular necrosis associated with mononuclear cells infiltration (Figure 5B), sinusoidal leukocytosis, and portal infiltration with mononuclear cells. Additionally, after 12 weeks of lead intoxication, liver showed hyperplasia of biliary epithelium and chronic cholangitis (Figure 5C). However, liver of rabbits cotreated with MOLEE and lead intoxication (4 weeks) showed an improved histopathological picture, and the examined sections revealed activation of Kupffer cells and slight cytoplasmic vacuolization of some hepatocytes (Figure 5D). Moreover, the liver of MOLEE-lead-intoxicated rabbits (8 weeks) revealed activation of Kupffer cells and slight hydropic degeneration of hepatocytes (Figure 5E). Examined sections from MOLEE-lead-intoxicated rabbits (12 weeks) showed congestion of central vein and hepatic sinusoids as well as vacuolar degeneration of hepatocytes (Figure 5F).
Concerning kidneys, examined sections from control
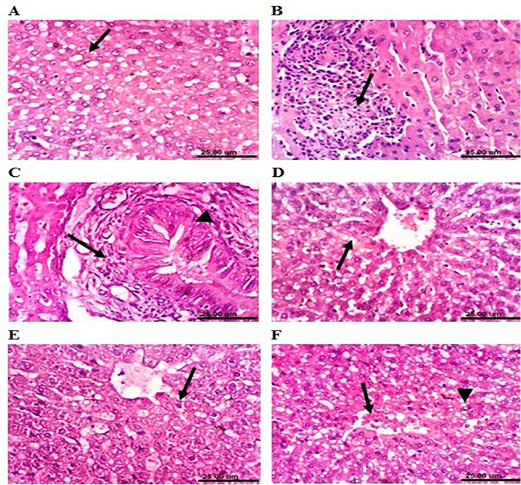
Figure 5: Photomicrograph sections from liver of a male rabbit. (A) Lead-intoxicated group (4 weeks) showing cytoplasmic vacuolization of hepatocytes (arrow). (B) Lead-intoxicated group (8 weeks) showing focal hepatocellular necrosis associated with inflammatory cell infiltration (arrow). (C) Lead-intoxicated group (12 weeks) showing hyperplasia of the biliary epithelium (arrowhead) and chronic cholangitis (arrow). (D) Moringa oleifera leaf ethanol extract- (MOLEE-) lead-intoxicated group (4 weeks) showing slight cytoplasmic vacuolization of hepatocytes (arrow). (E) MOLEE-lead-intoxicated group (8 weeks) showing slight hydropic degeneration of hepatocytes (arrow). (F) MOLEE-lead-intoxicated group (12 weeks) showing congestion of central vein (arrow) and vacuolar degeneration of hepatocytes (arrowhead). (H&E, scale bar: 25 µm).
rabbits revealed that renal parenchyma had normal histological structure of (Figure 6A). After 4 weeks, the kidneys of MOLEE-treated rabbits showed no histopathological alterations (Figure 6B). However, kidneys of MOLEE-treated rabbits (8 weeks) revealed vacuolation of epithelial lining renal tubules, hypertrophy, and vacuolation of glomerular tuft (Figure 6C). Furthermore, examined sections from MOLEE-treated rabbits (12 weeks) showed vacuolation of epithelial lining renal tubules, congestion of renal blood vessel (Figure 6D), and presence of proteinaceous material in the lumen of renal tubules.
On the other hand, kidneys of lead-intoxicated rabbits (4 weeks) revealed vacuolation of epithelial lining renal tubules and endothelial lining glomerular tuft (Figure 7A). Moreover, kidneys of lead-intoxicated rabbits (8 weeks) showed vacuolation of epithelial lining renal tubules and endothelial lining glomerular tuft as well as interstitial nephritis (Figure 7B). Kidney sections from lead-intoxicated
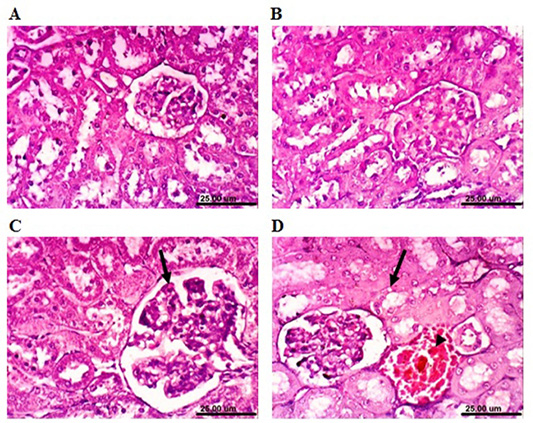
Figure 6: Photomicrograph sections from kidney of a male rabbit. (A) Normal control rabbits showing the normal histological architecture of renal parenchyma. (B) Moringa oleifera leaf ethanol extract- (MOLEE-) treated group (4 weeks) showing no histopathological alterations. (C) MOLEE-treated group (8 weeks) showing hypertrophy and vacuolation of glomerular tuft (arrow). (D) MOLEE-treated group (12 weeks) showing vacuolation of epithelial lining renal tubules (arrow) and renal blood vessel congestion (arrowhead). (H&E, scale bar: 25 µm).
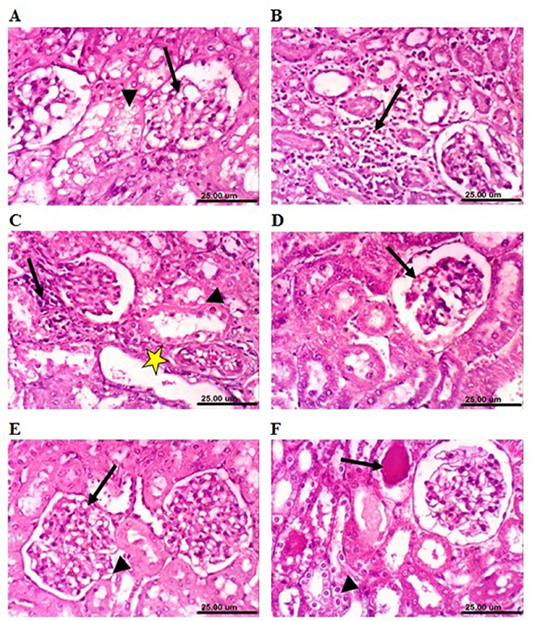
Figure 7: Photomicrograph sections from kidney of a male rabbit. (A) Lead-intoxicated group (4 weeks) showing vacuolation of epithelial lining renal tubules (arrowhead) and endothelial lining glomerular tuft (arrow). (B) Lead-intoxicated group (8 weeks) showing interstitial nephritis (arrow). (C) Lead-intoxicated group (12 weeks) showing vacuolation of epithelial lining renal tubules (arrowhead), focal necrosis of renal tubules (arrow), and cystic dilatation of renal tubules (yellow asterisk). (D) Moringa oleifera leaf ethanol extract- (MOLEE-) lead-intoxicated group (4 weeks) showing slight congestion of glomerular tuft (arrow). (E) MOLEE-lead-intoxicated group (8 weeks) showing slight vacuolation of epithelial lining renal tubules (short arrow) and endothelial lining glomerular tuft (arrow). (F) MOLEE-lead-intoxicated group (12 weeks) showing cytoplasmic vacuolation of epithelial lining renal tubules (short arrow) and eosinophilic proteinaceous cast in the lumen of renal tubules (arrow). (H&E, scale bar: 25 µm).)
rabbits (12 weeks) revealed vacuolation of epithelial lining renal tubules, focal necrosis of renal tubules, and cystic dilatation of renal tubules (Figure 7C). Meanwhile, kidneys of rabbits cotreated with MOLEE and lead intoxication (4 weeks) showed mild histopathological changes confined to slight vacuolation of epithelial lining of some renal tubules and slight congestion of glomerular tuft (Figure 7D). Furthermore, sections from MOLEE-lead-intoxicated rabbits (8 weeks) revealed congestion of glomerular tufts, with slight vacuolation of epithelial lining renal tubules and endothelial lining glomerular tuft (Figure 7E). Examined sections from MOLEE-lead-intoxicated rabbits (12 weeks) showed cystic dilatation of renal tubules, cytoplasmic vacuolation of epithelial lining renal tubules, and eosinophilic proteinaceous cast in the lumen of renal tubules (Figure 7F).
DISCUSSION
The present study was conducted to explore the role of MOLEE, as prophylactic treatment, in maintaining the structural cell integrity and protecting it from the adverse effects of lead-induced toxicity in rabbits. The effect of long-term MOLEE treatment on animal health was also discussed.
The obtained results showed that body weight gain markedly increased in MOLEE-treated rabbits. This may be attributed to the fact that Moringa oleifera leaves are a good source of protein, vitamins (vitamin C, eta-carotene), essential amino acids (methionine and lysine), and important macro- and microelements (calcium, potassium, iron, copper) (Ufele et al., 2013; Maizuwo et al., 2017). Lead-intoxicated rabbits showed a significant loss of body weight, which agrees with the findings by Khalil et al. (2018) who attributed the weight loss to the action of lead on the gastrointestinal tract, causing malabsorption of supplements and reduced food intake during a meal. In the present study, MOLEE slightly overcomes the action of lead intoxication on the weight gain of rabbits co-treated with MOLEE and lead intoxication. It could be due to the protection effect of MOLEE against lead-intoxication that resulted from its high nutritive values and bioactive substances (phytochemicals) contents (Lako et al., 2007; Maizuwo et al., 2017). The constant weight gain after 12 weeks may be attributed to a certain adverse effect that could happen due to the high content of phytochemical compounds (phenolics, tannins cumares, saponin, flavonoids, kaempferol, quercetin, alkaloids, phytate, and lectins) naturally occurring at high levels in Moringa oleifera leaves (Maizuwo et al., 2017; Atta et al., 2017).
In this study, the activity of ALT and AST markedly increased in MOLEE-treated group after 8 weeks of treatment. This could reflect the damage of hepatocytes. These results are well supported by the histopathological observation of liver sections that showed focal hepatic necrosis accompanied with inflammatory cells infiltration, cholangitis, and sinusoidal leukocytosis. Similar findings were recorded by Adedapo et al. (2009). Lead toxicity induced a significant increase in serum ALT and AST activities in the lead-intoxicated group. This finding is well correlated with the histopathological findings of liver sections, where focal hepatocellular necrosis associated with mononuclear cells infiltration was seen after 8 weeks of treatment. This result agrees with that by El-Tantawy (2016) who has attributed the results to the fact that the liver is one of the main organs engaging in storing, biotransforming, and detoxifying toxic substances, being thus susceptible to heavy metal poisoning. After 12 weeks of treatment, the increased serum AST activity in the MOLEE-lead-intoxicated group correlated well with the histopathology of the liver sections which displayed congestion of central vein and hepatic sinusoids as well as vacuolar degeneration of hepatocytes. During the periods of treatment, ALP activity markedly increased in the lead-intoxicated group. This could be due to biliary damage or obstruction of the biliary tree, which disturbs the blood flow to the liver (Farida et al., 2012). This finding is well correlated with the histopathological findings of liver sections, where hyperplasia of biliary epithelium and chronic cholangitis were seen after 12 weeks after lead intoxication. In previous studies, lead toxicity caused elevation of the activity of ALT, AST, and ALP in the blood serum of rats which resulted from hepatotoxic effects and was followed by intrahepatic cholestasis (Todorović et al., 2005; Herman et al., 2009). Serum ALT activity has been employed to assess liver necrosis (Bush, 1991). Increasing ALP activity could be an index of liver and bone damage which are correlated with the increased osteoblastic activity (Majkic-Singh, 1993). After 4 weeks of treatment, the ALP activity was restored to the normal level in MOLEE-lead-intoxicated rabbits, which might be due to the osteoprotective effect of MOLEE. Moringa oleifera has a miraculous effect on bone integrity and phytochemicals, particularly phytoestrogens which can have a positive impact on the bone, potently preventing osteoporosis (Burali et al., 2010).
In this study, MOLEE alleviated the action of lead acetate after 4 weeks in rabbits cotreated with MOLEE and lead intoxication. This could be due to the hepatoprotective effect of MOLEE which has potent antioxidant properties resulting from bioactive components (polyphenols, phenolic acids, flavonoids, alkaloids, glucosinolates, isothiocyanates, tannins, and saponins), vitamins, carotenoids, and macro- and microminerals (Atta et al., 2017; Vergara-Jimenez et al., 2017).
Concerning serum glucose in the lead-intoxicated group, the elevation in blood glucose levels may be due to altered DNA methylation. Mostafalou et al. (2015) referred to the possibility that the 0.05% lead concentration in potable water may cause intolerance of glucose and resistance to insulin in rats. Meanwhile, hypoglycemic activity in both MOLEE-treated and MOLEE-lead-intoxicated groups can be attributed to phytochemicals, bioactive compounds that have antioxidant properties correlated with a prophylactic activity against chronic degenerative diseases (Olayaki et al., 2015). This may explain the tendency of Moringa oleifera to reduce blood glucose levels in the present study, particularly in the MOLEE-lead-intoxicated group whose improvement was observed after 4 weeks compared to lead-intoxicated group. Moreover, the chlorogenic acid in the extract can favorably affect glucose metabolism and has been shown to inhibit glucose-6-phosphate translocase in the liver (each of the translocase subunits implements a particular function in the transport of substrates and products and eventually the release of glucose which will finally reach the bloodstream, as a step toward glycogenolysis or gluconeogenesis), reducing hepatic gluconeogenesis and glycogenolysis (Karthikesan et al., 2010). Olayaki et al. (2015) also observed that oral administration of MOLEE meaningfully lowers blood glucose concentration and prevents weight loss in alloxan-induced diabetic rats.
The level of serum blood urea and creatinine is known to reflect the state of glomerular filtration rate and kidney function (Kaneko et al., 2008). In the present work, lead-intoxicated rabbits showed that elevation of serum urea and creatinine corresponded well with the histopathology of the kidneys: congestion of glomerular tufts, slight vacuolation of epithelial lining renal tubules and endothelial lining glomerular tuft (after 8 weeks); focal necrosis of renal tubules, cystic dilatation of renal tubules (after 12 weeks). In this study, MOLEE alleviated the negative effect of lead acetate showing the absence of its effect on urea and creatinine in the MOLEE-lead-intoxicated group during the experimental periods. Prolonged administration of Moringa oleifera leaves as a beverage to Wister rats, in a dose-dependent manner, revealed significantly elevated total serum protein, globulin, blood urea, and creatinine which indicated liver and kidney damage (Omobowale et al., 2014). This might be the clue to the increased levels of urea and creatinine in MOLEE-treated group after 12 weeks in this study, confirmed by histopathological alterations such as vacuolation of epithelial lining renal tubules, congestion of renal blood vessel, and presence of proteinaceous material in the lumen of renal tubules. Furthermore, the results of this study agree with those by Awodelea et al. (2012), who revealed a slight increase in urea and creatinine levels in treated mice, confirming the need to use this medicinal herb with caution as it may cause long-term nephrotoxicity if consumed for a long time.
Regarding the total serum proteins, their increase after 12 weeks of treatment was a result of the increase in total globulins. These results agreed with those by Hristev et al. (2008), who reported that chronic treatment of the rabbits with lead and cadmium resulted in strong presence of hyper-beta-globulinemia with hypoalbuminemia of the background of normal proteinaemia or slightly hypoproteinemia. The decrease of serum albumin related to the degree of liver damage is higher. The improvement of total globulins has been noticed in the MOLEE-lead-intoxicated group after 12 weeks posttreatment compared to the lead-intoxicated group, which could be a result of antioxidants and hepatoprotective effect of MOLEE on liver tissue (Moyo et al., 2012).
In the present study, TC, TG, and LDL-C showed a significant increase while HDL-C showed a significant decrease in lead-intoxicated rabbits, which agrees with a previous study by Mohamed et al. (2020). HDL-C helps to scavenge cholesterol from extrahepatic tissues, and the reduction in the concentration of HDL-C in our study raised the TC levels. The association of increased serum TC and LDL-C levels with a higher risk for coronary heart diseases has been proved (Newairy and Abdou, 2009). Indeed, the increased cholesterogenesis found in the plasma of the lead-intoxicated rabbits may be due to lead toxicity-induced activation of 3‐hydroxy‐3-methylglutaryl coenzyme A (HMG-CoA) reductase and HMG-CoA synthase (the two rate-limiting enzymes in cholesterol synthesis), or it may be due to feedback inhibition (Sawada et al., 2005). The elevation of cholesterol above the normal values in lead-treated rabbits indicated one of the following: enhanced biosynthesis of cholesterol by hepatic cells; reduced hepatic reuptake of these molecules from the circulation by endocytosis mediated by a receptor (Tietz, 1999). Exposure to lead acetate may have caused oxidative stress as a result of the oxidation of cellular molecules comprising lipid and cholesterol, such as cell membrane phospholipids, lipoproteins, glycolipids, cholesterol, and other lipid-containing structures (Haleagrahara et al., 2011).
As seen in this study, Moringa oleifera contains phytosterols such as beta-sitosterol (Jain et al., 2010), and this compound can diminish intestinal uptake of dietary cholesterol (Liu et al., 2010). Moreover, the leaf consumption impact on the levels of triglycerides tends to lessen in healthy animals, which agrees with other studies that reported the lipid-lowering potential of Moringa oleifera (Chinedu et al., 2013; Atta et al., 2018; Mohamed et al., 2020). MOLEE reduced TC and LDL-C by inhibiting cholesterol synthesis and promoting bile acid synthesis by cholesterol. Other researchers have reported cholesterol-lowering potency in rats and rabbits fed with different parts of Moringa oleifera: crude leaf extract and cooked fruit (Mehta et al., 2003). This could partly account for the reduction in TC in this study. The pronounced decrease in TC, TG, and LDL-C obviously resulted in improvement in the MOLEE-lead-intoxicated group compared to the lead-intoxicated group.
The current data showed that lead toxicity may modify the redox status of the cell. MDA is a by-product of lipid peroxidation, which is an indicator of the degree of cell damage (Liu et al., 2010). During the lead treatment period (8 weeks), markedly increased MDA level and decreased GSH level were found in the liver tissue of the lead-intoxicated group. This could be due to the mechanism of lead-induced oxidative stress in tissues and cellular components which enhances free radical generation or depletes antioxidant enzyme system by causing peroxidative damage to membranes, DNA, proteins, and lipids (El-Tantawy, 2016; Mohamed et al., 2020). This study agreed with that of Offor et al. (2017) who stated that lipid peroxidation, which is another index of oxidative stress, results from the ROS activity on lipid membranes.
In the present study, the improvement effects of MOLEE on lead toxicity may refer to its antioxidative potential. Moringa oleifera is believed to be one of the main herbal remedies that play an important role in scavenging or inhibiting free radical production, reducing lipid peroxidation intensity, and boosting antioxidant enzyme activities, which is required to help cells survive exceptional environmental circumstances (Maduka et al., 2014).
The NO level markedly went up in lead-intoxicated rabbits. This could be due to lead that can interfere with the production of NO and can disrupt the function of nitric oxide synthase. This reflects NO synthase activity (Fidrianny et al., 2018). Meanwhile, MOLEE ameliorated the lead toxicity effect by lowering the NO level and restored it to the normal in MOLEE-lead-intoxicated rabbits. This could be due to the fact that MOLEE contains antioxidants (Atta et al., 2017) which have strong inhibitory effect on the production of NO in cells (Barbosa et al., 2006; Sierra-Campos et al., 2018). Similar findings were reported by Wahyuningsih et al. (2020) in lead-intoxicated mice treated with okra pods extract.
Regarding the negative changes in tissues and some of the biochemical parameters of liver and kidneys after 8 and 12 weeks in MOLEE-treated group, despite some improvements in MOLEE-lead-intoxicated group, Asiedu-Gyekye et al. (2014) confirmed the necessity of avoiding the possible cumulative toxicity of some of the MOLEE elements during long-term use. Further toxicological studies on Moringa oleifera from different places are needed in order to provide a reference regarding their relative safety and micro- and macroelemental composition.
Additionally, the improvement in MOLEE-lead-intoxicated group rather than MOLEE-treated group might be due to the direct effect of lead on the gastrointestinal tract, resulting in malabsorption of nutrients (Mohamed et al., 2016). According to Mobarak and Sharaf (2011), the toxic effects of lead were related to the intestinal damage including fusion of intestinal microvilli, necrosis and irregularities of the microvilli cells, microvilli loss, flattening, and hypertrophy. They inferred that exposure to lead acetate caused serious histopathological alterations in digestive organs of silver mollies. This could be the reason for decreasing the cumulative toxic effect of MOLEE, as the effect of lead acetate decreased its absorption. In addition, the potential antioxidant effects of MOLEE have been raised, which has been reflected in the improvements observed in the MOLEE-lead-intoxicated group.
Conclusions
Moringa oleifera has a preventive and protective effect on some biochemical and histological alterations induced by lead toxicity in rabbits. However, the highest performance did not continue during the experiment period in some parameters, and the beneficial effects of the plant began to decrease showing some adverse effects.
Recommendations
Results obtained according to working conditions of the present experiment revealed that Moringa oleifera has beneficial effects in increasing body weight. In addition, Moringa oleifera has a curing effect on lead toxicity, so it is recommended for humans and animals in polluted areas and in stress conditions. Nevertheless, this study made it clear that Moringa oleifera has restrictions in long-term use, so further studies are required to determine the safety of Moringa oleifera to avoid overdoses especially when it is used for extended time.
Acknowledgments
The authors are greatly indebted to Prof. Dr. Aboelfetoh M. Abdalla, Horticulture and Crops Technology Department, National Research Centre, Egypt for identification and supplying the plant material.
Authors’ contributions
AHM designed and supervised this study. NBM, NAA, SAN and SMN performed the extraction of M. oleifera ethanol and experiment, analysis of the blood and biochemistry, as well as data collection, analysis and interpretation. KAA examined and wrote the results of the histopathological samples. AHM, NBM, NAA, and SMN implemented writing the manuscript. All authors revised the manuscript for publication. All authors read and approved the final version of the manuscript.
REFERENCES