Advances in Animal and Veterinary Sciences
Research Article
Critical Factors Affecting the Quality of the Longissimus Lumborum from Native Thai Cattle (Bos indicus)
Ari Wibowo1*, Suhardi1, Khoiru Indana1, Krishna Purnawan Chandra1, Manat Chaijan2, Zuraida Hanum3
1Department of Animal Science, Faculty of Agriculture, Mulawarman University, Pasir Balengkong Rd, Gunung Kelua Campus, Samarinda, East Kalimantan, Indonesia, 75123; 2Food Technology and Innovation Research Center of Excellence, Division of Agro-Industry, School of Agricultural Technology, Walailak University, Nakhon Si Thammarat, Thailand 80161; 3Departement of Animal Science Faculty of Agriculture, Syiah Kuala University, Aceh, Indonesia, 23111.
Abstract | Colour is one of the important parameters determining the purchasing decision of consumer and it’s also become a challenging errand for the meat industry. The colour of meat itself is administered by the concentration and redox stage of myoglobin which that myoglobin underwent denaturation and oxidation in the presence of external triggers such as pH and temperature. Hence this study aimed to investigate the effect of chilled storage on the physicochemical changes and oxidative deterioration of Longissimus lumborum and their interrelationships between these traits from native Thai cattle. Physicochemical changes and oxidative deterioration of the Longissimus lumborum from native Thai cattle (n=3 female, 36 months, live wt. 350 kg) during storage at 5ºC from day 0 to 7 was investigated. Muscle pH decreased (6.26±0.05 to 5.53±0.32) and expressible drip increased (9.74 to 20.66) during storage and were highly correlated (R2 = 0.97). Autooxidation of oxymyoglobin followed a first-order kinetic model as steaks became more discolored as the redness index (a*/b*) decreased over time (1.55±0.12 to 1.02±0.05). Lipid oxidation increased during storage, where the thiobarbituric acid reactive substances (TBARS) (0.98±0.02 to 1.25±0.01) and it was correlated with percentage metmyoglobin (R2 = 0.91). Longissimus lumborum from native Thai cattle developed PSE-like meat as it became paler (greater L* values) soft and exudative (greater values for expressible drip and cooking losses). Values for muscle hardness gradually declined during storage, indicating a greater tenderness after 7 days of aging. Therefore, it can be concluded that these data indicate that the meat from native Thai cattle improved by controlling the critical factors (muscle pH, expressible drip, cooling losses, meat color, and color stability) during rigor development and post-mortem storage.
Keywords | Longissimus lumborum, native Thai cattle, myoglobin, color stability oxidation, physicochemical properties
Received | August 15, 2021; Accepted | October 25, 2021; Published | December 01, 2021
*Correspondence | Ari Wibowo, Department of Animal Science, Faculty of Agriculture, Mulawarman University, Pasir Balengkong Rd, Gunung Kelua Campus, Samarinda, East Kalimantan, Indonesia, 75123; Email: ariwibowo@faperta.ac.id
Citation | Wibowo A, Suhardi, Indana K, Chandra KP, Chaijan M, Hanum Z (2022). Critical factors affecting the quality of the longissimus lumborum from native thai cattle (bos indicus). Adv. Anim. Vet. Sci. 10(1): 107-113.
DOI | http://dx.doi.org/10.17582/journal.aavs/2022/10.1.107.113
ISSN (Online) | 2307-8316; ISSN (Print) | 2309-3331
Copyright © 2022 Wibowo et al. This is an open access article distributed under the Creative Commons Attribution License, which permits unrestricted use, distribution, and reproduction in any medium, provided the original work is properly cited.
INTRODUCTION
Thailand imported about 1570 tons of beef with an estimated value of 4.4 million USD from 1999 to 2006, and the amount tended to increase annually (Waritthitham et al., 2010). Consumer demand for high-quality beef has increased markedly, which has resulted in improvements in the domestic beef supply due to the development of local biodiversity efforts in a variety of production and animal husbandry practices (Samootkwam et al., 2015; Jaturasitha et al., 2009). However, to attain eminence of beef quality, additional refinements in the livestock and meat production chain are taking a systems approach involving effective animal breeding systems, animal health strategies, appropriate regional nutrition schemes, exceptional attention to antemortem handling and humane delivery of livestock to plants, and a continuum of postmortem practices for handling of carcasses and meat post-slaughter. Those will enhance beef quality and reduce or eliminate the downgrading of carcass meat and meat products. Special attention is being placed on sanitation and cold-chain management that effectively interfaces with economic benefits to both the beef industry and small meat retailers.
Quality characteristics of meat are influenced by various intrinsic and extrinsic factors, such as antemortem stress, muscle structure and its chemical composition, and the postmortem handling and storage conditions (Xiong et al., 1999). In the past, native Thai beef was sold fresh, without aging and with limited chilling, which made it difficult for retailers to maintain the desired eating quality of beef for at least a week. In daily practice, lipid oxidation, myoglobin redox instability, and the development of off-flavors and odors are a reality caused by inadvertent temperatures during storage (Ferguson et al., 2001; Olivera et al., 2013). Temperature control during storage is the most critical factor to control to prevent undue deterioration of enzymatic, chemical, physical, and microbial factors (Martin et al., 2013).
There is limited information about color and oxidative stability during chilled storage of the Longissimus lumborum, a preferred cut of beef (strip loin) from native Thai cattle. This study aimed to investigate the effect of chilled storage on the physicochemical changes and oxidative deterioration of Longissimus lumborum and their inter-relationships between these traits from native Thai cattle.
MATERIALS AND METHODS
Design Experiment and Meat Samples
A quasi-experiment was conducted to investigate the quality of Longissimus lumborum of Thai native beef cattle (Kamphaeng Saen beef cattle). The samples were obtained from three female Thai native beef cattle (Bos indicus- Kamphaeng Saen) which was being raised and farmed by local farmers and breeders, 36 months old and initial body weight of 350 ± 5.0 kg), were slaughtered and processed at a local commercial slaughterhouse in Thasala, Nakhon Si Thammarat, Thailand. After harvest, carcasses were fabricated within 3 h, and the retail cuts were consequently prepared without aging. Longissimus lumborum samples were collected and packed in plastic bags, covered with ice using an ice meat ratio of 2:1 (ice:meat, w/w) in the polystyrene box and transported to the Department of Agro-Industry, Walailak University within 30 min. Upon arrival, all of the visible fat and epimysia connective tissues were trimmed. Samples were cut into 2.54 cm thick steaks (150 g and substantially perpendicular to the fiber alignment) and packaged in polyethylene film in plastic trays. The pre rigor, packaged samples were placed in a cooler (5C) about 4 h post-harvest and stored in the dark (except for short times to gather samples) for 7 days. A single factor (storage days) experimet arranged in Completely Randomized Design using was applied in this experiment. During storage, three replicate samples were randomly taken for analyses at days 0, 2, 3, and 7 for physicochemical parameters, e.g., pH, colour, myoglobin redox, Thiobarbituric acid reactive substances (TBARS), expressible drip, cooking loss, and hardness.
Data Analysis
Data were subjected to analysis of variance. A comparison of the means was carried out by Duncan’s multiple range test to identify significant differences (P<0.05) among treatments. The data analysis was performed using the Statistical Package for Social Science (SPSS 10.0 for windows, SPSS Inc., Chicago, IL, U.S.A.). The statistical analysis was performed using SPSS v.23 to determine the kinetic model of the parameter changes and the interrelationship between the parameters, respectively.
Assays
Measurement of pH: Samples were homogenized using an IKA Labortechnik homogenizer with 10 volumes of deionized water (w/v), and the pH was measured using a calibrated (pH 4 and 7 buffers) pH meter following the methodology described by Chaijan et al. (2004).
Colour measurement: The surface color of Longissimus lumborum was analyzed using a portable Hunterlab Miniscan/EX instrument (Hunter Assoc. Laboratory; VA, USA) 10 standard observer, Illuminant D65, and aperture size of 2.54 cm. Tristimulus values (average of 2 scans/sample) L* (lightness), a* (redness/greenness), and b* (yellowness/blueness) values were reported. The redness index (a*/b*) of samples were determined as described by Chen et al. (1997).
Myoglobin redox and the kinetics of oxymyoglobin oxidation: Measurement of myoglobin redox forms was conducted using methods described by Faustman and Philips (2001). Meat samples (25 g) were homogenized for 30-45 sec with 75 ml of 40 mM sodium phosphate buffer (pH 6.8). The homogenate was filtered through double-layer cheesecloth, and the absorbance was recorded from 500 to 600 nm using the buffer as blank. The proportions of the oxymyoglobin and metmyoglobin were calculated using Krzywicki’s equations as modified by Tang et al. (2004).
[Oxymyoglobin] = 0.722 R1 - 1.432 R2 - 1.659 R3 + 2.599
[Metmyoglobin] = -0.159 R1 - 0.085 R2 + 1.262 R3 - 0.520
Note: R1 = A582/A525, R2 = A557/A525 and R3 = A503/A525.
The oxymyoglobin autoxidation rate over time was determined by calculating the loss of oxymyoglobin using the formula from Tajima and Shikama (1987), as follows:
Autoxidation rate = Ln([oxymyoglobin]t/[oxymyoglobin]0)
where [oxymyoglobin]t is the fraction of oxymyoglobin at time t, and [oxymyoglobin]0 is the fraction of oxymyoglobin at the start of the experiment (day 0).
Measurement of thiobarbituric acid reactive substances (TBARS)
The TBARS assay was performed as described by Buege and Aust (1978). A ground sample (0.5 g) was homogenized by potter homogenizer in a 2.5 mL solution containing 0.375% (w/v) thiobarbituric acid, 15% (w/v) trichloroacetic acid and 0.25 N HCl. The mixture was heated in a boiling water bath (95-100 C) for 10 min to develop a pink color, then cooled with running tap water and centrifuged at 3,600 g at 25 C for 20 min. The absorbance of the supernatant was measured at 532 nm. A standard curve was prepared using 1,1,3,3-tetra methoxy propane at concentrations ranging from 0 to 10 ppm. TBARS was calculated and expressed as equivalents of mg malonaldehyde (MDA)/kg meat sample.
Expressible drip determination
Expressible drip was measured according to the method of Ng (1987). Samples, 0.05 cm in thickness, were weighed and placed on top of three pieces of filter paper (Whatman paper no. 1), and two pieces of the same type of filter paper were placed on top of the two samples. A standard weight (5 kg) was placed on the top of the sample and maintained for 2 min. The samples were removed and re-weighed. Expressible drip was calculated and expressed as a percentage of the initial sample weight.
Cooking loss determination
Cooking loss was calculated as a percentage of the difference in the weight of raw and cooked samples divided by the raw weight times 100, as described by Purslow et al. (2016). Meat samples were cut along their long axis parallel to the muscle fiber direction, and the raw weight was recorded. Afterward, samples were placed individually in separate plastic bags and heated simultaneously in a temperature-controlled water bath (Memmert, Schwabach, Germany) set at 75 °C for 60 min. After heating, samples were transferred to an ice bath and cooled for 15 min, lightly patted dry, and their weights measured to determine the percentage of weight lost during cooking. The average of 2 samples was used for statistical analyses.
Hardness determination
Texture analysis of meat was performed using a TA-XT2 texture analyzer (Stable Micro Systems, Godalming, Surrey, U.K.). Samples, 2.54 cm, was cut from the middle of the LL muscle, which was used for taking the cores (3 cut sizes x 3 muscles), were prepared and assigned to trial per cooking loss determination each day. Hardness was measured using the texture analyzer equipped with a spherical plunger (diameter 5 mm; depression speed 60 mm/min) and expressed as newton (Onega et al., 2005).
RESULT AND DISCUSSION
The interrelationship between expressible drip and muscle pH
Figure 1 shows the relationship between Longissimus lumborum muscle pH and expressible drip of Thai native beef during chilled storage for 7 days. The pH values decreased before adequate chilling, which contributed to greater expressible drip throughout cold storage. The initial pH value of Longissimus lumborum from Thai native beef was 6.26, and it declined to 5.53 at storage day 7. Expressible drip increased from 9.74% to 20.66% on day 7. Postmortem pH has major effects on the water holding capacity of meat during cold storage (Lawrie and Ledward, 2006), and the pH will continue to decrease during storage as long as glycogen is adequate Zybert et al. (2014).
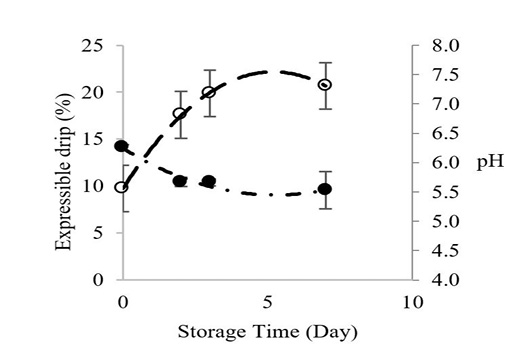
Figure 1: Changes inexpressible drip (○) and pH () of Longissimus lumborum from Thai native cattle during chilled storage (15 oC). Bars indicate standard deviation from triplicate determinations. Pearson Product-Moment Correlation between the two parameters is 0.9695.
Theoretically, drip loss is strongly influenced by an interaction of a low muscle pH and a too-warm, pre-rigor muscle temperature that can denature proteins, lighten the color and result in a lower-than-normal, post-rigor pH that is closer to the muscle’s isoelectric point (pI). The lowest water holding capacity occurred when the pH was equal or close to pI. Generally, the pI of myosin is about 5.3-5.5 (Huff-Lonergan and Lonergan, 2005). This combination of postmortem events before the meat is chilled causes a reduction of electrical charges on muscle proteins, which is associated with a shrinkage of the myofibrillar lattice and a reduction in space between myofibrils; hence water is released (Huff-Lonergan and Lonergan, 2005). Our pH and expressible drip data were highly correlated (R2 = 0.97), which agrees with Otto et al., 2006 who reported increased water losses starting 2 to 3 days through day 7 of cold storage.
The interrelationship between myoglobin oxidation, lipid oxidation and discoloration of meat
Changes in oxymyoglobin and metmyoglobin contents in Longissimus lumborum from Thai native cattle during chilled storage are shown in Figure 2. Oxymyoglobin significantly decreased by the time of storage (P<0.05). After 7 days of chilled storage at 15oC, the oxymyoglobin decreased by 33.09 % when compared with the initial value (day 0).

Figure 2: Changes in oxymyoglobin autooxidation rate () and percentage of TBARS increased (■) (a) and changes in redness index (b) of Longissimus lumborum from Thai native cattle during chilled storage. Bars indicate standard deviation from triplicate determinations. Pearson Product Moment Correlation between the redness index and autooxidation of oxymyoglobin is 0.8516.
A decrease in oxymyoglobin content in Longissimus lumborum from Thai native cattle during storage could be influenced by several factors such as the decreases of oxygen diffusivity and oxygen consumption of meat (Beriain et al., 2009; Mohan et al., 2010), the inactivation of metmyoglobin reductase and the depletion the endogenous antioxidants (Yu et al., 2017). Yu et al. (2017) reported that oxymyoglobin content in Psoas major declined continuously with prolonged storage. For metmyoglobin content, it gradually increased with increasing chilled storage time (P<0.05). In the end, metmyoglobin increased by 64.60% when compared with day 0, corresponding with the marked oxidation of oxymyoglobin. The result was similar to a previous study of Beriain et al. (2009), who found the increase in metmyoglobin during chilled storage of Longissimus lumborum from Pirenaica bulls.
Color is one of the critical parameters determining the purchasing decision of the consumer. In a fresh meat, myoglobin be able to in 3 different redox states; deoxymyoglobin, oxymyoglobin, and metmyoglobin where the oxymyoglobin state is responsible for the purchaser-preferred bright cherry-red color as a fresh meat. Moreover, Heme is an iron-containing compound of the porphyrin class which forms the nonprotein part of myoglobin which also has an important role to affect the state of myoglobin redox or changing color and discoloration in meat. The color of meat is governed by the concentration and redox state of myoglobin (Beriain et al., 2009) caused by the presence of external triggers such as pH and temperature Mukherjee et al. (2017).
Reactive species, including superoxide anion, hydrogen peroxide, and hydroxyl radical, can be generated during oxymyoglobin oxidation (Chaijan, 2008). Ultimately, the hydroxyl radical penetrates and immerses into the hydrophobic area of lipid and can induce lipid oxidation (Chaijan, 2008; Chan et al., 1997). It was well established that myoglobin can potentially stimulate lipid oxidation (Jie et al., 2017). The relationship between changes in oxymyoglobin, metmyoglobin, and TBARS during storage is presented in Figure 3.

Figure 3: Changes in oxymyoglobin content (○), metmyoglobin content (), and TBARS (■) of Longissimus lumborum from Thai native cattle during chilled storage. Bars indicate standard deviation from triplicate determinations. Pearson Product Moment Correlation between the formation of metmyoglobin and TBARS content is 0.9144.
The TBARS content and metmyoglobin formation increased steadily by the time of storage, and they were correlated well with the R2 value is 9144. However, after 7 days of storage, the Longissimus lumborum muscle of Thai native cattle showed a TBARS content of 1.34 mg MDA/kg meat, which was under the threshold of the off-odors and off-taste. Generally, the flavor and taste changes can be detected in meat and meat products when the TBARS content is equal to or more than 5 mg MDA/ kg meat (Nakyinsige et al., 2014).
The oxymyoglobin and lipid oxidation increased during chilled storage, as shown by the oxymyoglobin autooxidation rate and the percentage of TBARS (Figure 3). Longissimus lumborum meat had a high elevation of oxymyoglobin autooxidation during the early storage (day 2-3), and it gradually increased at day 7 with the concomitantly increased of TBARS during storage. Chaijan (2008) reported a strong correlation between oxymyoglobin oxidation and lipid oxidation both in microsomes and liposomes. Oxymyoglobin autooxidation, together with the lipid oxidation, would enhance the discoloration of Longissimus lumborum from Thai native beef, as evidenced by the marked decrease of redness index during storage (Figure 2b). The loss of the redness index was correlated with the autooxidation of oxymyoglobin (R2 = 0. 8516).
Besides, the decrease in pH during storage may facilitate the oxidations of both myoglobin and lipid of Longissimus lumborum from Thai native beef. It could be seen the increases of oxymyoglobin and lipid oxidations with the gradual decrease in pH during storage. The result was consistent with previous research of Richards and Hultin (2000), who found that the autoxidation of the haem pigment occurred more rapidly at reduced pH. Yin et al. (2017) also reported the acceleration of lipid oxidation in beef, which was more rapid during iced storage at pH 6.3 compared to pH 6.7.
PSE-like phenomenon indicated by changes in L*, hardness and cooking loss
Interrelationships between L*, hardness, and cooking loss in Longissimus lumborum from Thai native cattle during chilled storage are shown in Figure 4. L* values and percentage cooking losses increased as hardness decreased, especially from storage days 3 to 7. Cooking losses also increased (P< 0.05) during chilled storage (Figure 4). A strong correlation, R2 = 0.90, occurred between hardness and percentage cooking loss and, when combined with higher L* values, support PSE-like muscle properties. When expressible juice and cooking loss data are combined and associated with hardness, these data suggest several avenues for improving the quality of beef from native Thai cattle.
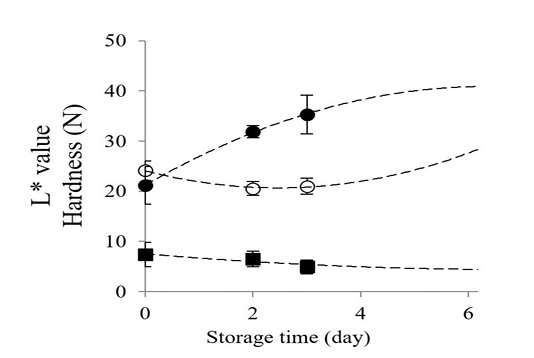
Figure 4: Changes in L* value (○), hardness (■), and cooking loss () of Longissimus lumborum from Thai native cattle during chilled storage. Bars indicate standard deviation from triplicate determinations.
From the results, the L* value tended to increase during storage gradually. After 7 days, the L* increased by 33.14% when compared with day 0. This finding showed consistent with previous research in which L* value reflected the purge of water in meat (Yu et al., 2017). Hardness tended to decrease during storage. After 7 days, hardness decreased by 40.2% compared to day 0, while the cooking loss increased markedly during chilled storage. In the end, cooking loss increased by 20.66% when compared to day 0. The high purge of water as results from expressible drip and cooking loss can affect the quality of beef, particularly the tenderness, color, and nutritional value of cooked meat. Loss of micronutrients with the cooking juice can alter the nutritional profile of end-products (Oillic et al., 2011).
CONCLUSIONS
Longissimus lumborum from Thai native cattle that had minimal carcass chilling combined with typical merchandising conditions of Thai small meat retailers underwent oxidative deterioration and physicochemical alteration during chilled storage. Myoglobin oxidation, lipid oxidation, and discoloration of meat were distinctly observed during storage, and they were interlinked among several traits. Strong correlations between muscle pH, expressible drip, cooling losses, meat color, and color stability were indicative that they are a candidate for critical factors that could be applied to improve overall longissimus quality, especially for Thai meat with PSE-like meat properties. Therefore, the further research is needed to address this aspect in future, especially on controlling the critical factors which contribute to discoloration and lipid oxidation in meat during cold storage.
Acknowledgments
This work was supported by Walailak University Fund.
Conflicts of interest
No potential conflicts of interest relevant to this article were reported.
authors contribution
Ari Wibowo: Overall conceptualization of the study, acquisition, analysis and interpretation of data, formulation of proposed strategies, manuscript writing, as well as finalization of edits and revisions necessary. Suhardi: Provided support in the conceptualization of the research design. Khoiru Indana: Provided substantial contribution to theory development and organization of data. Krishna Purnawan Chandral: Provided substantial contribution in organization of data and responsible for lipid oxidation and meat color (myoglobin redox) analysis. Manat Chaijan: Assisted in the conceptualization and streamlining of the research, contributed in the research design, performed data analysis and strategy development, critical evaluation of research data and content, fund and facility sourcing as well as checking for manuscript revisions necessary. Zuraida Hanum: Provided material discussion on theory development and critical evaluation of research data and content.
REFERENCES