Advances in Animal and Veterinary Sciences
Research Article
Detection of Meat Species Substitution in Some Ready to Eat Beef-Based Meat Products in Egypt
Bassant H. Elsheikh1*, Riyad R. Shawish2, Zakaria H. Elbayoumi2
1Veterinary Food Hygienist, University of Sadat City, Egypt; 2Department of Food Hygiene and Control, Faculty of Veterinary Medicine, University of Sadat City, Egypt.
Abstract | Hundred random samples of some ready to eat (RTE) beef-based meat products, represented by Kabab, sausage, kofta, burger, and Hawawshi (20 of each) each weighted about 250 gm, collected from random restaurants of variable hygienic levels, located in Cairo and Menoufiya governorates, Egypt; for detection of their fraudulent substitution with inexpensive, lower grade, or forbidden meats, and investigate its safety for human consumption depending on their bacteriological quality. Accordingly, all collected samples of meat products were subjected to certain techniques including glycogen test, precipitation test, Polymerase Chain Reaction (PCR) to confirm their safety for human consumption. Furthermore, the fractionation of amino acids and fatty acids was applied to differentiate between sound and adulterated meat products. Referring to the obtained results by glycogen (%), precipitation test, and PCR techniques; 18, 19, and 19% of the examined samples were recorded to be adulterated with meats other than beef meat, which were furtherly supported by positive amino acids and fatty acid fractionation for equine and canine meat adulteration. Furthermore, E. coli, Salmonella species, and Staphylococcus aureus were detected in 57.9, 26.3, and 47.4% of the examined positively recorded as adulterated samples to possess a serious food poisoning potential, consequently of a public health hazard. Conclusively, the current study had thrown light some fraud practices in meat products with illegal additions of equine and canine meats, besides that molecular technique revealed as a specific, sensitive, and rapid method for detection of meat species adulteration; moreover, it is worthly recommended authorities to apply strict supervision over food manufacturing facilities for safe and wholesome meat production.
Keywords | Species substitution, Ready-to-Eat, Meat products, Adulteration, Multiplex PCR
Received | May 11, 2021; Accepted | September 29, 2021; Published | December 01, 2021
*Correspondence | Bassant Hamdy Elsheikh, Veterinary Food Hygienist, University of Sadat City, Egypt; Email: [email protected]
Citation | Elsheikh BH, Shawish RR, Elbayoumi ZH (2022).Detection of meat species substitution in some ready to eat beef-based meat products in Egypt. Adv. Anim. Vet. Sci. 10(1): 68-77.
DOI | http://dx.doi.org/10.17582/journal.aavs/2022/10.1.68.77
ISSN (Online) | 2307-8316; ISSN (Print) | 2309-3331
Copyright © 2022 Elsheikh et al. This is an open access article distributed under the Creative Commons Attribution License, which permits unrestricted use, distribution, and reproduction in any medium, provided the original work is properly cited.
INTRODUCTION
Fast foods are ready to eat (RTE) foods that have been prepared and sold for direct consumption in public places. It has been supposed to supply safe, high-quality, and nutritional meals with reasonable prices, that assemble a major source of daily feeding of many consumers with a wide variety of meat products (Samapundo et al., 2015); but unfortunately, increased consumers’ demands encouraged greedy meat producers to adulterate meat products, especially finely cut and minced products.
Animal proteins, especially those from high quality meat sources, can attain high prices in the market, thus being prone to fraudulent substitutions, either by other lower value meat species (as occurred in the recent European horse meat scandal) or by vegetable proteins like soybeans (Soares et al., 2013).
The fraudulent substitution or adulteration of high-quality meat with its inferior/cheaper equivalent is a frequent practice in meat production globally including Egypt. The distinction of species meat origin is of great importance due to trading, wellbeing, and religious implications (Abd El-Aziz, 2009). In addition, accurate species identification of meat products is of special attention due to the emergence of zoonotic diseases through meat products.
As can be seen, obtaining a low-cost product is as simple as combining other proteins like vegetable and cheaper proteins of other species; nevertheless, meals incorporating pig origins are Haram (unlawful or banned) for Muslims to ingest. Furthermore, a comparison of pig and other red meat sources reveals that pork has less nutritional value than the others due to the destruction of Vitamin-E. It’s also been shown that because pork has a high risk of illness, it exacerbates health issues. Furthermore, utilizing chemicals in excess of the allowed amounts poses a significant public health risk. Referring to records, more than 25% of ready-to-eat meat products are mislabeled. Another recent example of adulteration is the miss nomenclature of meat products constituents which has a negative impact on manufacturers and results in unfair competition (Kozan et al., 2013).
Several techniques already exist for the verification of the meat origin in meat products, which are based on organoleptic and chemical properties of meat, but unfortunately, each has its restrictions. The physicochemical methods, like glycogen percent test, are expressive only in raw untreated meats, so they are not useful in ground meats; the immunological techniques, like precipitation test, are also considered to have complicated isolation of species-specific proteins process; in addition, the used antisera may show cross-reactions especially post-cooking; furthermore, these methods are also laborious and of inferior sensitivity at a lower level of adulteration, and are qualitative only (Luo et al., 2008; Rout et al., 2018).
Recently, DNA extraction techniques have been used for species specification in meat products depending on their several advantages; from which genetic material is uniquely present in specific cell types with identical information, regardless of the sample origin. In addition, preparation and molecular examination of meat extracted DNA is highly reasonable and extremely stable especially in heat-treated meat products. DNA-based analyses are prevalent regarding particularity, precision, reliability, and lawful agreeableness (Meira et al., 2017).
The role of food microbiology is to study the food microbial ecology, sources and probability of causing food poisoning. Furthermore, because of their capacity to cause illnesses, some microbes pose a significant threat to public health as had been recorded like Escherichia coli, Staphylococcus aureus and Salmonella species which contribute a worldwide public health issue (Húngaro et al., 2014).
Thus, there is considerable interest and need, not only for wholesome meat production and processing but also for the identification of meat origin, to certify meat product quality and protect consumers from being a victim of fraudulent adulteration. Therefore, this investigation aimed to determine the incidence of beef substitution in some beef-based RTE meat products represented by kabab, sausage, kofta, burger, and shawarma, which were collected from Cairo and Menoufiya governorates, with equine, canine, and pork meats depending on glycogen percent, precipitation, and molecular techniques; moreover, its bacteriological quality was assessed.
MATERIALS AND METHODS
Collection of samples
One hundred different samples of RTE beef-based meat products represented by Kabab, sausage, kofta, burger, and hawawshi (20 of each) were collected from variable restaurants of variable hygienic levels located in Cairo and Menoufiya governments, Egypt. Each sample was wrapped separately and transferred to the laboratory for the detection of their substitution with forbidden meats of other animal species.
All samples were subjected to glycogen test, precipitation test, PCR and fractionation of amino acids and fatty acids to differentiate between sound and adulterated meat products.
Glycogen test (Pearson, 2006)
Accurately, 20g of the examined samples were minced and digested by an 80 ml alcoholic solution of potassium hydroxide (10%) until all tissues are dissolved. The mixture was filtrated and neutralized. The glucose content was estimated by using a spectrophotometer (Spectronic 21). Further, the glycogen % was calculated by the following formula:
Glycogen% = glucose % × 0.927
Precipitation test (ppt)
The technique recommended by Mackie and McCartney (1996) was carried out for detection of adulteration of the examined meat products with prohibited meat; using patent specific antisera (Sigma-Aldrich Chemie Gmb, 82024 Taufkirchen, Germany) for different animals including beef (B3759), equine (H8890), dog (D4908) and pig (P3164) meats were used in the present work.
Polymerase chain reaction (PCR)
Genomic DNA extraction was performed using a commercial Gene JET genomic DNA purification kit (Mag Pure DNA LQ Kit D6312-01, Bioteck Angen, Yayingshi Road, Luogang District, Guangzhou, China), which was mixed with PCR master Mix (Fermentis) for amplification. Finally, the amplified product was separated and electrophorized according to Sambrook et al. (1989). DNA extracts were examined in The Central Laboratory of Food Research, Faculty of Veterinary Medicine, Benha University, Egypt.
Primer sequences of cytB of cattle, equine, and pork species-specific genes for PCR identification system were documented in Table 1.
Primer sequences of cytB of the canine species-specific gene for the PCR identification system were documented in Table 2.
DNA amplification
Target genes, amplicons sizes, and cycling conditions were documented in Table 3.
Agarose gel was prepared in percentage of 1.5% with ethidium bromide solution (10 mg/ml) in Tris borate EDTA (TBE) electrophoresis buffer (1x). A 100bp DNA ladder was used.
Fractionation of amino acids
The technique recommended by Mabbott (1990) for fractionation of amino acids was applied by Gas-Liquid Chromatography (GLC).
Fractionation of fatty acid
After extraction of fat from the examined samples according to Aura et al. (1995), fatty acids were determined by the Gas Chromatography technique (GC) according to AOAC (2006).
Bacteriological examination
Detection and identification of enteropathogenic E. coli
After preparation of serial dilutions of each sample according to ISO 6887-1 (2013), E. coli was detected according to ISO 16649-2 (2001) serotyped using diagnostic E. coli antisera (SSI DIAGNOSTICA).
Detection and identification of Salmonella species was performed according to ISO 6579 (2017) and serologically (Diep et al., 2019).
Detection and identification of S. aureus according to ISO 6888-1(1999).
After preparation of the sample according to ISO 6887-1 (2013), Baird-Parker agar plates were inoculated by surface spreading technique and incubated at 37oC for 48h, followed by biochemical identification as recommended.
Statistical analysis
The overall collected data were evaluated by application of Analysis of Variance (ANOVA) test using SPSS software V.20 according to Armstrong et al. (2000).
Results and Discussion
Glycogen %
Glycogen % was used to indicate adulteration of the examined meat products, especially with equine meats. As recorded in Table 3, a total of eighteen samples showed a higher percent of glycogen content than the maximum permissible limits (MPL) (MPL= <0.25%), from which hawawshi revealed the highest prevalence of adulterated product (30%). In addition, statistical analysis of variance proved significant differences between the examined samples when (p≤0.05).
Precipitation test (PPT)
Referring to the ppt results (Table 4), 19% out of the examined samples showed adulteration with other meat species; from which seven samples were revealed to be pure equine meat, while 10, and 2 samples revealed intermixed with equine and canine meats, respectively; in addition, 81 samples revealed pure beef meat as recorded in Table 5.
Molecular detection of meat product authentication
Referring to molecular detection of cytB genes of the tested species as demonstrated in Figures 1, 2, and Table 6, it came to confirm precipitation test results and proved that, 19 (19%) samples showed adulteration with other meat species, represented by 7 samples revealed as pure equine meat, 10 samples revealed intermix beef with equine meats, furthermore, two samples revealed detection of canine cyt B gene. While 81 samples revealed pure beef meat.
Table 1: Primer sequences of cytB of cattle, equine and pork species-specific genes for PCR identification system.
Spp. | Target gene |
Oligonucleotide sequence (5′ → 3′) |
Product size (bp) | References |
SIM* |
cyt b (F) |
5′ CCTCCCAGCTCCATCAAACATCTCATCTTGATGAAA′3 |
Matsunaga et al. (1999)
|
|
Cattle |
cyt b (R) |
5′ CTAGAAAAGTGTAAGACCCGTAATATAA′3 |
274 | |
Equine |
cyt b (R) |
5′ CTCAGATTCACTCGACGAGGGTAGTA ′3 |
439 | |
Pig |
cyt b (R) |
5′ GCTGATAGTAGATTTGTGATGACCGTA ′3 |
398 | |
canine |
cyt b (F) |
5′ GGAGTATGCTTGATTCTACAG ′3 |
808 | Abdulmawjood and Buetter (2003) |
cyt b (R) |
5′ AGAAGTGGAATGAATGCC ′3 |
Table 2: Target genes, amplicons sizes and cycling conditions.
References | Final extension | Amplification (31 cycles) |
Primary denaturation |
Amplified segment (bp.) | Target gene | Species | ||
Extension | Annealing | Secondary denaturation | ||||||
Jain et al. (2007) |
72˚C 10 min. |
72˚C 30 sec. |
60˚C 30 sec. |
94˚C 30 sec. |
94˚C 5 min. |
274 bp |
cyt b (R) |
Cattle |
439 bp |
cyt b (R) |
Equine | ||||||
398 bp |
cyt b (R) |
Pig | ||||||
Amplification (35 cycles) | ||||||||
Edris et al. (2012) |
72˚C 10 min. |
72˚C 60 sec. |
58˚C 60 sec. |
94˚C 60 sec. |
94˚C 4 min. |
808 bp |
cyt b (F) |
Dog |
Table 3: Detection of adulteration in the examined samples of meat products based on their Glycogen % (n=20).
Meat products |
Min | Max | Mean ± S.E. | Adulterated samples* | |
No. | % | ||||
Kabab | 0.11 | 1.43 |
0.31 ± 0.01 ed |
2 | 10 |
Burger | 0.16 | 1.49 |
0.37 ± 0.02 d |
3 | 15 |
Kofta | 0.16 | 1.57 |
0.42 ± 0.02 c |
3 | 15 |
Sausage | 0.18 | 1.60 |
0.50 ± 0.03 b |
4 | 20 |
Hawawshi | 0.19 | 1.64 |
0.61 ± 0.05 a |
6 | 30 |
Total (100) | 18 | 18 |
* The glycogen % in beef should not exceed 0.25% Pearson (2006). (a, b, c, d, ed) values within a column with different superscript letters were significantly different at (P ≤ 0.05).
Table 4: Percentages of adulteration the examined meat products with meat of other animal species using precipitation test (n=20).
Meat product | Adulterated | Non-adulterated | ||
No. | % | No. | % | |
Kabab | 2 | 10 | 18 | 90 |
Burger | 3 | 15 | 17 | 85 |
Kofta | 3 | 15 | 17 | 85 |
Sausage | 5 | 25 | 15 | 75 |
Hawawshi | 6 | 30 | 14 | 70 |
Total | 19 | 19 | 81 | 81 |
Table 5: Interpretation of precipitation test for detection of adulteration in the examined samples of meat products (n=20).
Meat product |
Cattle | Equine | Cattle and equine | Cattle and Dog | Cattle and Pig | |||||
No. | % | No. | % | No. | % | No. | % | No. | % | |
Kabab | 18 | 90 | 2 | 10 | 0 | 0 | 0 | 0 | 0 | 0 |
Burger | 17 | 85 | 0 | 0 | 3 | 15 | 0 | 0 | 0 | 0 |
Kofta | 17 | 85 | 1 | 5 | 2 | 10 | 0 | 0 | 0 | 0 |
Sausage | 15 | 75 | 2 | 10 | 2 | 10 | 1 | 5 | 0 | 0 |
Hawawshi | 14 | 70 | 2 | 10 | 3 | 15 | 1 | 5 | 0 | 0 |
Total (100) | 81 | 81 | 7 | 7 | 10 | 10 | 2 | 10 | 0 | 0 |
Table 6: Interpretation of PCR technique for detection of adulteration in the examined samples of meat products (n=20).
Meat product | Kabab | Burger | Kofta | Sausage | Hawawshi | |||||
No | % | No | No | No | % | No | % | No | % | |
Pure cattle meat | 18 | 90 | 17 | 85 | 17 | 85 | 15 | 75 | 14 | 70 |
Pure equine meat | 2 | 10 | 0 | 0 | 1 | 5 | 2 | 10 | 2 | 10 |
Cattle and equine meat | 0 | 0 | 3 | 15 | 2 | 10 | 2 | 10 | 3 | 15 |
Cattle and dog meat | 0 | 0 | 0 | 0 | 0 | 0 | 1 | 5 | 1 | 5 |
Pig meat | 0 | 0 | 0 | 0 | 0 | 0 | 0 | 0 | 0 | 0 |
Total (100) | 20 | 100 | 20 | 100 | 20 | 100 | 20 | 100 | 20 | 100 |
Table 7: Fractionation of amino acid composition (%) in the examined meat product samples.
Amino acids | Cattle | Equine | Cattle and equine | Cattle and dog | Pig |
1. Essential A.As: | |||||
Arginine | 6.1 | 4.8 | 5.3 | 4.9 | 5.8 |
Histidine | 2.6 | 2.3 | 2.5 | 2.2 | 3.1 |
Iso-leucine | 4.9 | 4.1 | 4.6 | 3.8 | 5.2 |
Leucine | 8.7 | 9.3 | 8.9 | 8.1 | 7.4 |
Cystine | 1.4 | 2.7 | 2.0 | 1.6 | 1.3 |
Lysine | 7.9 | 6.6 | 7.1 | 7.4 | 6.1 |
Methionine | 2.1 | 3.4 | 2.8 | 1.7 | 2.6 |
Phenylalanine | 3.9 | 2.5 | 3.1 | 3.8 | 3.4 |
Threonine | 4.4 | 3.1 | 3.3 | 4.0 | 5.6 |
Tryptophan | 1.2 | 1.5 | 1.4 | 1.1 | 1.6 |
Valine | 5.7 | 5.1 | 5.5 | 4.6 | 5.0 |
Total | 48.9 | 45.4 | 46.5 | 42.2 | 47.1 |
2. Non Essential A.As: | |||||
Serine | 4.1 | 4.8 | 4.6 | 3.1 | 4.9 |
Aspartic acid | 8.7 | 9.6 | 9.1 | 5.8 | 9.0 |
Glutamic acid | 14.6 | 15.1 | 14.9 | 16.2 | 15.4 |
Glycine | 7.0 | 7.4 | 7.1 | 7.9 | 6.3 |
Proline | 5.3 | 4.6 | 4.8 | 7.1 | 4.6 |
Alanine | 6.2 | 7.3 | 6.8 | 6.6 | 5.7 |
Tyrosine | 3.5 | 2.9 | 3.2 | 4.7 | 2.8 |
Total | 49.4 | 4.8 | 50.5 | 54.4 | 48.7 |
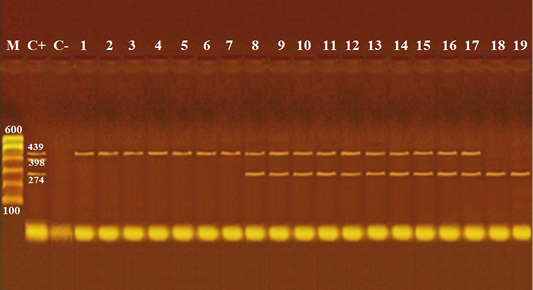
Figure 1: Agarose gel electrophoresis of multiplex PCR of cytB gene of cattle (274 bp), pig (398 bp) and equine (439 bp) for detection of adulteration of meat products.
Lane M: 100 bp ladder as molecular size DNA marker; Lane C+: Control positive for cyt b gene of cattle, pig and equine meat; Lane C-: Control negative; Lanes 1 and 2: Pure Equine meat detected in kabab; Lanes 3: Pure Equine meat detected in kofta; Lanes 4 and 5: Pure Equine meat detected in sausage; Lanes 6 and 7: Pure Equine meat detected in hawawshi; Lanes 8, 9 and 10: Cattle meat intermixed with equine meat detected in burger; Lanes 11 and 12: Cattle meat intermixed with equine meat detected in kofta; Lanes 13 and 14: Cattle meat intermixed with equine meat detected in sausage; Lanes 15, 16 and 17: Cattle meat intermixed with equine meat detected in hawawshi; Lanes 18 and 19: Cattle meat free from equine meat detected in sausage.
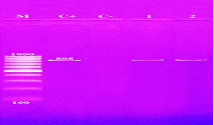
Figure 2: Agarose gel electrophoresis of PCR for detection of adulteration of meat products with dog meat using cytB gene of dog (808 bp).
Lane M: 100 bp ladder as molecular size DNA marker; Lane C+: Control positive for cyt b gene of dog meat; Lane C-: Control negative; Lane 1: Dog meat detected in sausage; Lane 2: Dog meat detected in hawawshi.
Amino acids and fatty acids fractionation
Additionally, amino acids (AA) and fatty acids (FA) were fractioned as tabulated in Table 7. Referring to results of AA fractions, leucine, cystine, methionine, serine, and aspartic acid ratio indicated substitution with equine meat; while detection of glutamic acid, glycine, proline, alanine, and tyrosine indicated adulteration with canine meats. Also, saturated and unsaturated FA (Table 8) fractions played a great role in species identification and detection of species adulteration.
Moreover, out of the examined samples, pork meat was not positively detected neither by ppt nor PCR detection of meat species adulteration.
Bacteriological quality of the positively adulterated samples
Consequently, adulterated samples were furtherly assessed for their bacteriological profile of some food poisoning bacteria, Table 9 illustrated detection of E. coli in 11/19 (57.9%). Moreover, Salmonella spp. was detected in 5/19 (26.3%). In addition, S. aureus was detected in 9/19 (47.4%) out of the examined samples; where hawawshi samples showed the highest prevalence of bacterial contamination, followed by sausage, kofta, burger and kabab, respectively.
Meat adulteration, especially with species substitution, is a global complication that contravenes food codes. Thus, detection of the species of the meat components in meat products is an essential task in food hygiene, especially in further processed products, meat adulteration in advanced ground and processed products has been a widespread problem in retail markets.
In Egypt, all the examined meat products including kabab, sausage, kofta, burger, and hawawshi dishes are common RTE meat-based products, and generally prepared from cattle meats. Essentially, they are flavorful, ready-to-eat meat products that are usually prepared fresh and served hot as part of splendid meals. The demand for such ready-to-eat meat products is increasing day by day mainly due to socio-economic development and changing lifestyles. As meat and meat products represent an important and large component of human food, their quality is of concern to the consumers, the regulatory authorities, the processors, and the retailers.
In the present study, higher glycogen (%) than MPL was recorded in 10, 15, 15, 20, and 30% of the examined kabab, burger, kofta, sausage, and hawawshi samples, respectively. Previous studies were conducted in Egypt, the current results came in line with the recorded results by El-Shewy (2007) who recorded adulteration in 8% and 12% of the examined kabab and kofta samples collected from Qalubiya governorate, while higher prevalence was detected in the same samples which were collected from Gharbia governorate (36 and 40%, respectively); in addition, Abd El-Sadek (2011) findings came in agree with the present prevalence of hawawshi adulteration (30%), while higher prevalence was detected in the burger (25%), and kofta (20%), but the lower result was recorded in sausage samples (15%) collected from Cairo and Giza governorates; moreover, Etawy (2018) recorded a higher prevalence of glycogen level in the examined sausage samples (33.3%) collected from Menoufiya governorate, Egypt.
Glycogen percent is usually used as an indicator of meat species adulteration depending on the muscular glycogen content, which is varying depends on the age, the species, fattening conditions, and the pre-slaughter state of the food animal (Gallo, 2003).
Referring to the obtained results of precipitation (ppt) test, the present records revealed that 10, 15, 15, 25, and 30% of the examined kabab, burger, kofta, sausage, and hawawshi were considered to be adulterated; from which 7, 10 and 10% were of pure equine meat, intermixed with equine and canine meats, respectively.
Table 8: Fractionation of fatty acid composition (%) in the examined meat product samples.
Fatty acids | Cattle | Equine | Cattle and equine | Cattle and dog | Pig |
Lauric acid (C12:0) | 0.1 | ND | ND | 0.2 | ND |
Myristic (C14:0) | 0.4 | 0.2 | 0.3 | 0.7 | 0.1 |
Palmitic (C16:0) | 21.4 | 23.7 | 22.5 | 25.2 | 17.2 |
Stearic (C18:0) | 13.5 | 14.8 | 13.9 | 14.0 | 12.3 |
Total Saturated F.As | 34.9 | 38.5 | 36.7 | 40.1 | 29.6 |
Palmitoleic (C16:1) | 1.9 | 1.1 | 1.6 | 1.5 | 2.5 |
Oleic (C18:1) | 23.7 | 21.9 | 23.1 | 19.8 | 16.9 |
Total Mono-Unsaturated F.As | 25.6 | 23.0 | 24.7 | 21.3 | 19.4 |
Linoleic (C18:2) | 17.8 | 16.5 | 17.1 | 14.9 | 24.7 |
Linolenic (C18:3) | 0.6 | 0.8 | 0.6 | 0.2 | 1.2 |
Eicosadienoic acid (C20:2) | ND | 0.1 | 0.1 | ND | 0.3 |
Dihomo-γ-linolenic (C20:3) |
0.1 | 0.1 | 0.1 | ND | 0.2 |
Arachidonic (C20:4) | 10.3 | 9.4 | 9.8 | 8.7 | 9.9 |
Eicosapentaenoic “EPA” (C20:5) | 0.1 | ND | ND | ND | ND |
Docosapentaenoic “DPA” (C22:5) | 0.2 | ND | 0.1 | 0.3 | ND |
Docosahexaenoic “DHA” (C22:6) | ND | ND | ND | 0.1 | 0.1 |
Total Poly-Unsaturated F.As | 29.1 | 26.9 | 27.7 | 24.2 | 36.3 |
Table 9: Incidence of food poisoning bacteria isolated from the examined samples of adulterated meat products (N=19).
Meat product | Kabab (n=2) |
Burger (n=3) |
Kofta (n=3) |
Sausage (n=5) |
Hawawshi (n=6) |
Total (N=19) |
||||||
Isolated pathogens | No. | % | No. | % | No. | % | No. | % | No. | % | No. | % |
E. coli O17:H18 |
1 | 50* | ND | - | ND | - | ND | - | ND | - | 1 | 5.3** |
E. coli O91:H21 |
ND | - | ND | - | 1 | 33.3* | 2 | 66.6* | 2 | 66.6* | 5 | 26.3** |
E. coli O114:H4 |
ND | - | ND | - | ND | - | ND | - | 2 | 66.6* | 2 | 10.5** |
E. coli O121:H7 |
ND | - | ND | - | ND | - | ND | - | 1 | 5.3* | 1 | 5.3** |
E. coli O127:H6 |
ND | - | ND | - | ND | - | 2 | 66.6* | ND | - | 2 | 10.5** |
Total | 1 | 5.3** | ND | - | 1 | 5.3** | 4 | 21.1** | 5 | 26.3** | 11 | 57.9** |
Salmonella Enteriditis | ND | - | ND | - | ND | - | 1 | 20.0* | ND | - | 1 | 5.3** |
Salmonella Essen | ND | - | ND | - | ND | - | 1 | 20.0* | ND | - | 1 | 5.3** |
Salmonella Typhimurium | ND | - | ND | - | ND | - | 2 | 40.0* | 1 | 16.6* | 3 | 15.8** |
Total | ND | - | ND | - | ND | - | 4 | 21.1* | 1 | 5.3* | 5 | 26.3** |
Staphylococcus aureus | 1 | 50* | 1 | 33.3* | 2 | 66.6* | 2 | 40* | 3 | 50* | 9 | 47.4** |
Total | 1 | 5.3** | 1 | 5.3** | 2 | 10.5** | 2 | 10.5** | 3 | 15.8** |
N. total number of the recorded adulterated samples (19). n, number of the recorded adulterated samples for each meat product. *, Prevalence of the microorganism in relation to the recorded number of the adulterated samples of each examined meat product. **, Prevalence of the microorganism in relation to the total recorded number of the adulterated samples (19).
lower results were previously recorded by El-Shewy (2007) who declared detection of equine meat in 4 and 8 of the examined kabab and kofta samples collected from Qalubiya governorate, while higher prevalence was recorded in the same samples collected from Gharbia governorate (28 and 32%, respectively); in addition, Abd El-Sadek (2011) recorded higher ppt findings in 45, 30, 35, and 40% of the examined burger, sausage, kofta, and hawawshi samples with meat other than beef species, respectively. Moreover, Etawy (2018) recorded adulteration of meat species in a total of 4(26.67%) of the examined sausage samples, where one sample was intermixed with equine meats, while the other three samples revealed that their meat was of pure equine meats; furthermore, out of 15 examined sausage samples, dog meat was not detected by ppt test.
Precipitation test or known as Ring Precipitation Test (RPT) in which homologous antigen and antibodies reacts together and make a ring at the point of its interaction. Known antibodies (blood serum) collected from the treated experimental animals are mixed in a test tube with the filtered extract of meat samples. If precipitation line forms at the point of mixing then it indicates a positive test. But it has some limitations such as this test can apply only for qualitative evaluation, with some shortage in its application for heat-treated meat. Besides that, it varies according to individual variations and cross-reactivity (Singh and Neelam, 2011). Therefore, DNA-based molecular techniques are of choice for species specifications due to their stability during heating and processing, high sensitivity and specificity, as well as expeditious processing time, and low cost.
Molecular detection of cytB gene of different examined meat species as shown in Table 7 and Figures 1, 2 revealed detection of pure equine meat in 5% of the examined kofta samples, and 10% of the examined kabab, sausage, and hawawshi samples of each in addition, 15% of burger and hawawshi of each, and 10% of kofta and sausage samples of each were of cattle meat intermixed with equine meat; moreover, canine meat appeared in 5% of sausage and hawawshi of each as intermixed with cattle meats. While pork meat was not detected in any of the examined samples.
Previous authors used the PCR technique for species authentication such as Abd El-Sadek (2011), Hassanin et al. (2018), and Hamouda et al. (2020) who investigated species adulteration with other than beef meats in a burger, kofta, and sausage samples, where results revealed negative detection of equine and/or porcine meats, while canine meat was detected in 33.3 and 66.7% of the examined kofta and sausage samples, respectively. In addition, Khatun et al. (2021) recorded examination of 64 samples of RTE sausage, kabab, burger, and meatball in Bangladesh by PCR technique, where results revealed that the examined samples were free from pork meats, and concluded that species‐specific mitochondrial CytB gene primers and found to be accurate for species identification in meat and milk products.
Amino acids are the base bricks compounds of all proteins of any living organism. Amino acids are divided into essential, partially interchangeable, and interchangeable. Essential amino acids are not synthesized in the human body but are necessary for its normal life, so they must be consumed with foods. A lack of essential amino acids delayed the growth and development of the body. Interchangeable amino acids are synthesized in the human body. For humans, the main source of amino acids is foods rich in protein like meat and meat products (Zaretsky and Wreschner, 2008).
The obtained results of AA and FA fractionation gave a broad view over the specific structure of meats of different species regarding it as a significant tool of meat species adulteration based on the characterized concentration of each acid in comparison to standard values.
Alekseeva and Kolchina (2019) recorded that the main detected amino acids composition of Aberdeen-Angus cattle breed were valine, histidine, leucine, isoleucine, lysine, threonine, phenylalanine, methionine, tryptophan, alanine, aspartic acid glycine, glutamic acid, serine, tyrosine, hydroxyproline, arginine, and cystine, it agreed with the conclusion of Edris et al. (2012) who recommended the application of amino acids and fatty acids fractionation for detection of meat substitution by other animal tissues and to ensure its compliance with legal and compositional standards of some meat products.
Although ready to eat meat products cover a wide part of human meat consumption, they have been also identified as one of the commonest sources of foodborne disease outbreaks. Studies revealed that each year foodborne microbial pathogens cause illnesses, which affect 6 to 80 million persons in the United States, causing 9,000 deaths mainly due to foodborne infection with E. coli, Salmonella, and S. aureus bacteria (CDC, 2010). Therefore, the present study performed a bacteriological assessment of the adulterated samples. E. coli was detected in 57.9% of the examined samples, where serological identification revealed isolation of E. coli O17:H18, O91:H21, O114:H4, O121:H7, O127:H6. In addition, Salmonella species was detected in 26.3% of the examined samples, where serological identification revealed isolation of S. Enteriditis, S. Essen, and S. Typhimurium. Moreover, Staphylococcus aureus was detected in 47.4% of the examined samples.
Nearly similar results were recorded by Hazaa (2015) (who detected nearly similar prevalence of S. aureus (52.2%) in the total eighty examined samples of burger, kofta, sausage, and hawawshi, while lower prevalence of E. coli (20%) and Salmonella (15%) were recorded); Rawash (2015) (recorded higher results of S. aureus in 60 and 46.6% of the examined kofta and burger samples, respectively, while E. coli was detected in 33.3 and 26.27%; in addition, Salmonella was detected in 26.7 and 13.3% of the same samples, respectively), and Gaafar (2020) (who detected S. aureus in 40% of the examined kofta samples, while lower prevalence of E. coli and Salmonella were recorded (10% and 6.66%, respectively).
Referring to the bacteriological examination of the positively recorded as a species adulterated samples, high prevalence of such food poisoning bacteria in the indicated serious issue, not only as species substitution but also possess a public health threat.
Such detected pathogens were incriminated in many serious foodborne outbreaks, KDHE (2019) recorded that more than 100 serovars of E. coli produce systemic and enteric toxins. In the U.S., it is estimated that 70,000 infections per year were caused by E. coli O157. Persons of all ages were susceptible, especially of low or incompletely developed immunity, showing severe illness and HUS. Furthermore, S. aureus has the potential to cause a variety of infections accompanied by challengeable treatment referring to its ability to form biofilms and the emergence of multidrug resistance in addition, staphylococcal enterotoxins possess a great challenge in the food safety field (Oliveira et al., 2018). Furthermore, IDSC (2014) reported that S. Enteritidis had been recorded to be the most prevalent serovar since 1989 among the reported human Salmonellosis in Japan; moreover, between 2010-2014, S. Enteritidis came among the most common Salmonella serovars detected in human outbreaks in Japan.
Conclusion and Recommendations
Conclusively, the examined hawawshi samples revealed the highest incidence of meat-species adulteration and bacterial profile as well, followed by sausage, while the examined kofta and burger samples showed nearly similar results in relation to meat-species substitution and bacterial profile; while kabab samples revealed the lowest prevalence of adulteration and bacterial contamination. Meat product adulteration by species meat substitution revealed to be a serious widespread problem with health hazard concerns, especially when accompanied by bacterial food poisoning. So, PCR showed to be the most beneficial, rapid, reliable, and sensitive detection technique. Therefore, the application of regular screening of meat species adulteration commercially is strongly recommended to safeguard consumer’s health.
Acknowledgments
We would like to express our special thanks to all members of the Food Hygiene and Control Department, Faculty of Veterinary Medicine, University of Sadat City, Egypt for their valuable help and encouragement.
Novelty Statement
Authentication and prevalence of fraudness by illegal beef meat substitution with other forbidden meat species were investigated in ready to eat kabab, burger, kofta, sausage and hawawshi meals in Egypt chemically by glycogen percent test, immunologically by precipitation test, and molecularly by PCR technique. Moreover, bacteriological quality of the positively frauded samples was assessed for determining its safety.
Author’s Contribution
BHE collected samples from different markets and restaurants, performed the practical part of the research including physicochemical, immunological and molecular assays; performed the required statistical tests and typed them in tables, typed the manuscript in this form, and uploaded and followed-up the research publication. RRS and ZHE develop the research plan, and supervise its implementation. All the entire authors reviewed the research before publication.
Conflict of interest
The authors have declared no conflict of interest.
References